Pii: s0925-3467(99)00119-6
Optical Materials 14 (2000) 101±107
Optical properties of lissamine functionalized Nd3 complexes
in polymer waveguides and solution
L.H. Sloo a,*, A. Polman a, S.I. Klink b, G.A. Hebbink b, L. Grave b,
F.C.J.M. van Veggel b, D.N. Reinhoudt b, J.W. Hofstraat c
a FOM Institute for Atomic and Molecular Physics, Kruislaan 407, 1098 SJ Amsterdam, The Netherlands
b Supramolecular Chemistry and Technology, University of Twente, P.O. Box 217, 7500 AE Enschede, The Netherlands
c Philips Research, Department of Polymers and Organic Chemistry, Prof. Holstlaan 4, 5656 AA Eindhoven, The Netherlands
Received 3 May 1999; accepted 5 November 1999
Lissamine functionalized terphenyl-based Nd complexes are synthesized, and incorporated in deuterated dimethyl-
sulfoxide solutions and partially ¯uorinated planar polymer waveguides. Optical excitation of the lissamine sensitizer
around 500 nm, followed by intramolecular energy transfer to the Nd3 ion, causes near-infrared photoluminescence
(890, 1060, 1340 nm) due to intra-4f transitions in the Nd3 ion. The intramolecular energy transfer rate is larger than
107 sÿ1. Due to the large absorption cross-section of the sensitizer (>10ÿ17 cm2 around 500 nm), the Nd3 is excited 104
times more eciently than in a pure complex, without sensitizer. The Nd3 luminescence lifetime is relatively short,
both in solution (2.2 ls) and in a polymer host (0.8 ls), which is attributed to coupling to vibrational states of nearby
C±H and O±H groups. Spincoated ¯uorinated polymer planar waveguides, doped with these sensitized organic Nd
complexes show excellent waveguide properties. Upon continued illumination, photodegradation is observed in the
doped polymer ®lms. Ó 2000 Elsevier Science B.V. All rights reserved.
the parity forbidden transitions slightly allowed.
The lifetimes of these transitions are therefore
Trivalent rare earth ions are well known for
relatively long.
their special optical properties [1]. The 4f-shell of
These properties make the rare earth ions useful
these ions is not completely ®lled and shielded
for applications in integrated optics. The rare
from the surroundings by ®lled 5s and 5p shells.
earths erbium (Er) and neodymium (Nd) are
This shielding minimizes the eect of the crystal
commonly used in optical ®ber ampli®ers [2±5] due
®eld of the host material on the energy levels of the
to their intra-4f transitions at 1550 nm (Er,
4f-shell and as a result the absorption and emission
4I13=2 ! 4I15=2) and 1340 nm (Nd, 4I3=2 ! 4I11=2),
bands remain rather sharp. The small in¯uence of
two standard telecommunication wavelengths. A
the crystal ®eld induces mixing of wave functions
lot of research has focused on rare earth-doped
with opposite parity within the 4f-shell, making
solid-state planar optical ampli®ers for integrated
optics applications, and working planar ampli®ers
have been reported for rare earth ions in silica,
* Corresponding author.
Al2O3, phosphate glasses, and LiNbO3 [6,7]. The
0925-3467/00/$ - see front matter Ó 2000 Elsevier Science B.V. All rights reserved.
L.H. Sloo et al. / Optical Materials 14 (2000) 101±107
growing importance of polymer thin ®lms in inte-
grated optics technology makes it interesting to
study rare earth doped polymer waveguides, and
see if polymer optical ampli®ers can be made [8,9].
These polymer waveguide ampli®ers could then be
integrated with existing polymer devices such as
splitters, switches, and multiplexers [10].
Rare earth ions cannot be dissolved directly
into a polymer ®lm. Therefore, the ions have to be
encapsulated by an organic ligand to form a
complex, which can be dissolved in the polymer
matrix. Previously, we have shown that optically
Fig. 1. Schematic energy level diagram of the lissamine±Nd3
active Er-doped polydentate cage complexes can
complex. The arrows indicate the excitation mechanisms of the
be synthesized, and show room temperature pho-
Nd3 ion: either directly into the 4G7=2 level by pumping at 515
nm, or through the lissamine sensitizer (S
toluminescence at 1.535 lm when optically excited
0 ® S1 transition fol-
lowed by intersystem crossing and energy transfer).
either directly into an Er level, or indirectly via the
cage which also acts as a chromophore [11]. We
found that the luminescence lifetime of these
tizer, the 515 nm light is mainly absorbed by the
complexes is rather short (0.8 ls), which was at-
high absorbing lissamine, which becomes excited
tributed to energy transfer of the excited state of
into the singlet state (S1). This is followed by
the Er3 ion to ±OH and ±CH vibrational states of
intersystem crossing to the triplet state (T,
the complex or of the solvent molecules [12,13].
ET 14600 cmÿ1) (see Fig. 1). From the triplet
Deuteration of ±CH and ±OH bonds reduces some
state energy transfer to the Nd3 ion can occur,
of these quenching paths [14].
which results in excitation of the Nd3 ion into the
Obviously these non-radiative quenching pro-
4S3=2 and 4F9=2 levels. After relaxation to the 4F3=2
cesses are a disadvantage of the use of organic cage
level the 890, 1060 and 1340 nm luminescence can
complexes. On the other hand, an advantage is
be observed. The luminescence intensities, life-
that highly absorbing antenna chromophores can
times, excitation mechanisms, waveguide proper-
be incorporated in the organic complex. Once this
ties, and photostability of these complexes will be
chromophore is excited it can transfer its excita-
tion energy to the rare earth ion. If the energy
transfer from chromophore to rare earth ion is
ecient, this process strongly enhances the exci-
tation eciency of the rare earth ion.
In this paper, we will report the optical prop-
Terphenyl-based Nd3 complexes were synthe-
erties of terphenyl-based Nd3 complexes with and
sized [15] using the procedure described in Ref.
without a highly absorbing lissamine antenna
[15]. Some complexes were functionalized with
chromophore. Complexes were dissolved either in
lissamine, a Rhodamine-B derivative [16]. Fig. 2
hexadeutero-dimethylsulfoxide (DMSO-d6) solu-
shows a schematic picture of the structure of the
tions or in partially ¯uorinated polycarbonate
terphenyl-based Nd3 complexes (a) with two
planar waveguides. In complexes without lissa-
benzoyl side-groups (Bz.Nd) and (b) with one
mine, excitation of the Nd3 ion at a wavelength of
benzoyl side-group and a lissamine sensitizer
515 nm leads to population of the 4G7=2 level, from
(Ls.Nd). Both complexes have a cage-like con®g-
where it decays to the 4F3=2 level (see Fig. 1). De-
uration, encapsulating the Nd3 ion. The com-
cay from this level leads to the characteristic Nd3
plexes were dissolved in DMSO-d6 to a
luminescence at 890, 1060 and 1340 nm due to
concentration of 10ÿ2 M for Bz.Nd and 10ÿ6 M for
transitions to the 4I9=2, 4I11=2 and 4I13=2 levels, re-
Ls.Nd, or dissolved in partially ¯uorinated poly-
spectively. In complexes with the lissamine sensi-
carbonate [17] waveguides at a concentration of
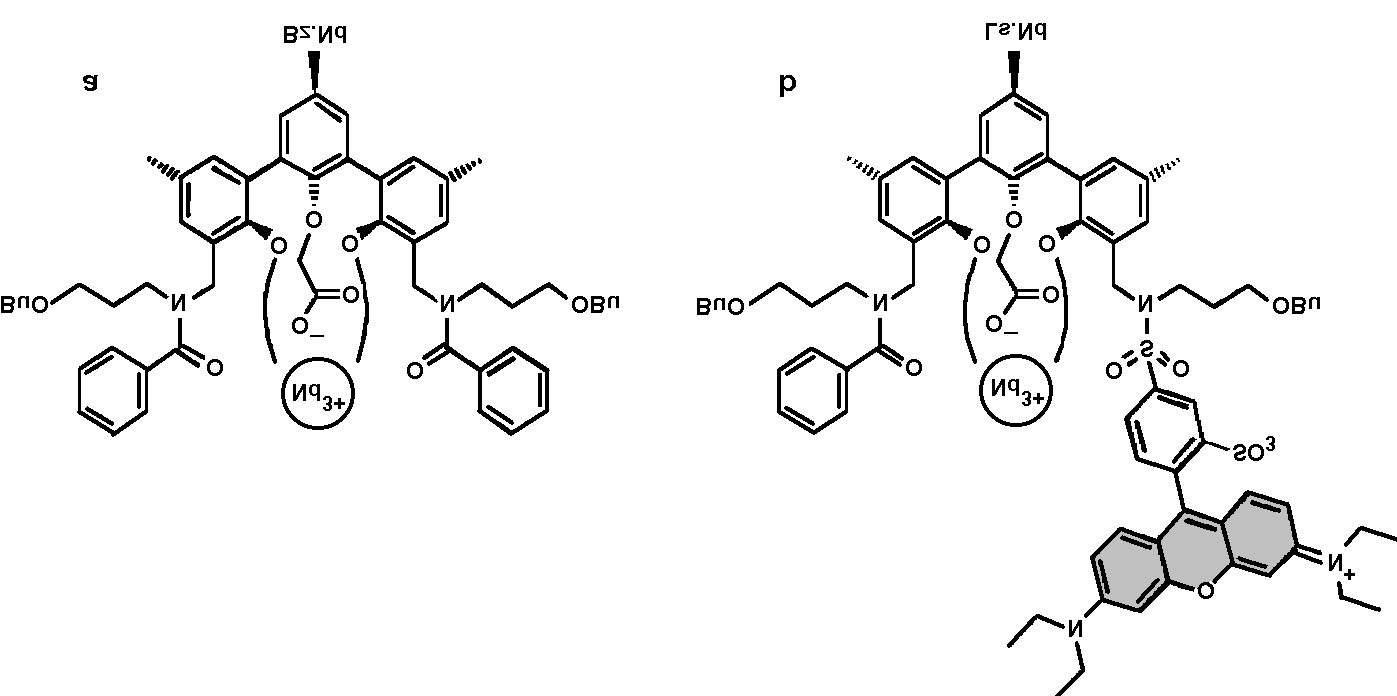
L.H. Sloo et al. / Optical Materials 14 (2000) 101±107
Fig. 2. Schematic picture of (a) benzoyl±Nd3 complex (Bz.Nd) and (b) lissamine functionalized Nd3 complex (Ls.Nd).
3 wt% (complex). The polycarbonate waveguides
the entrance facet of the waveguide. The lumines-
were made by spincoating a cyclohexylacetate so-
cence was collected at the output facet, using a
lution of polycarbonate and complex onto a Si
multi-mode optical ®ber. The ®ber was led to an
substrate covered with a 3 lm thick thermally
objective in front of the monochromator. The
grown SiO2 layer. The spincoating was performed
spectral resolution ranged from 0.2 to 6 nm.
for 30 s at a spinrate of 3000 sÿ1 and followed by
Photoluminescence decay curves were measured
thermal annealing at 190°C (in vacuum) for 1 h.
using a photomultiplier tube and a photon
The thickness of the polymer layer was 3.55 lm.
counting system. The time resolution of the system
The real and imaginary parts of the refractive
was about 100 ns.
index of the polymer waveguide were measured
using a variable angle spectroscopic ellipsometer.
The optical losses were measured using the sliding
3. Results and discussion
prism method [18]. Diiodomethane was used as an
index matching liquid for optimum output cou-
Fig. 3 shows the PL intensities at 1060 nm for a
pling. A white light source as well as lasers oper-
10ÿ2 M solution of Bz.Nd and a 10ÿ6 M solution
ating at 633, 838, 1305 and 1565 nm were used.
of Ls.Nd (lissamine sensitized), both in DMSO-d6,
Photoluminescence (PL) measurements were
at dierent excitation wavelengths as available
performed using various lines of an Ar-ion laser as
from the Ar-ion laser. The absorption spectrum
an excitation source. The complexes in DMSO-d6
measured for the same solutions is also included in
solution were analyzed in square quartz cells. The
the ®gure. The pump power for excitation was 60
power on the cell was 60 mW, at a spot diameter of
mW for all excitation wavelengths. The excitation
1 mm. The beam was modulated using an acousto-
spectrum for the complex without sensitizer
optic modulator, operating at dierent frequen-
(Bz.Nd) shows some structure, which is roughly
cies. The emitted luminescence was focused into a
similar to that found in the absorption measure-
monochromator and detected with a photomulti-
ment, and is consistent with the absorption bands
plier tube or a liquid-nitrogen-cooled Ge detector.
of the Nd3 ion around 475 and 513 nm. The ex-
All spectra were corrected for the detector re-
citation spectrum for the complex with sensitizer
sponse. Absorption measurements were performed
(Ls.Nd) shows a completely dierent behavior: the
using a spectrophotometer. Measurements on
1060 nm emission intensity increases strongly with
waveguide ®lms were performed by pumping the
excitation wavelength, again very similar to what
waveguide (total length about 25 mm) with a
is found for the absorption spectrum. Given the
rectangular spot
5 15 mm from the top near
fact that the lissamine complex shows a broad
L.H. Sloo et al. / Optical Materials 14 (2000) 101±107
temperature PL of Nd3 at 890, 1060, and 1340
nm. Although the concentration of Ls.Nd is 104
times lower than the concentration of Bz.Nd, the
PL intensity is two times higher. Optical absorp-
tion measurements at 515 nm for both solutions
show an almost equal absorption: 0.033 cmÿ1 for
10ÿ2 M Bz.Nd and 0.031 cmÿ1 for 10ÿ6 M Ls.Nd.
The fact that the sensitized complex shows higher
luminescence than the complex without a sensi-
tizer, even though the measured absorption was
the same, indicates that the internal energy trans-
fer eciency within the sensitized complex is quite
Fig. 3. Photoluminescence at 1060 nm as a function of excita-
high. The factor 2 dierence can be due to the fact,
tion wavelength for Bz.Nd (10ÿ2 M, squares) and Ls.Nd (10ÿ6
M, circles) in DMSO-d6 solutions. The absorption spectra of
that upon direct excitation into the higher lying
Bz.Nd and Ls.Nd are also shown (drawn lines). The absorption
state of the Nd3 ion, the Nd3 ion can also decay
data for the Bz.Nd solution are multiplied by a factor of 2000.
radiatively to the ground level (indeed, 524 nm
luminescence has been observed, resulting from
the transition from the 2K
absorption band around 580 nm, this clearly in-
13=2 ! 4I9=2 transition),
leading to a lower quantum yield for the near-in-
dicates that the excitation of Nd3 around 500 nm
frared transitions in the case of direct optical ex-
takes place via the sensitizer. The absorption of the
citation of the Nd3 at 515 nm. The measured
lissamine occurs at the xanthene unit (i.e. the gray
luminescence lifetime (not shown) at 1060 nm for
part in the structure for Ls.Nd in Fig. 2(b)). Note
the Bz.Nd complex in DMSO-d6 is 2.5 ls, and for
that the measured absorption cross-section is in
the sensitized Ls.Nd complex it is 2.2 ls. The lu-
the 10ÿ17 cm2 range, which is four orders of mag-
minescence lifetime of Nd3 in inorganic materials
nitude above the typical Nd3 intra-4f transition
can be as high as 250 ls [19]. The low quantum
yield in the organic complexes is attributed to
Fig. 4 shows the room-temperature PL spectra
quenching of the Nd3 excited state by coupling to
for 10ÿ2 M Bz.Nd and 10ÿ6 M Ls.Nd in DMSO-
overtones of nearby C±H and O±H vibrational
d6, recorded using excitation at 515 nm at a pump
power of 60 mW. The complexes show room-
Fig. 5(a) shows the measured (dashed line) and
simulated (solid line) ellipsometry parameter D as
a function of wavelength for a partially ¯uorinated
polycarbonate planar polymer waveguide doped
with 3 wt%. Ls.Nd. The interference structure is
caused by re¯ections at the air/polymer, polymer/
SiO2, and SiO2/Si interface. A clear dip in D is
observed around 580 nm, which is caused by the
high absorption of the lissamine. The simulated
data are based on a Lorentz oscillator model and
correspond well with the measured data. From the
simulation parameters, the real (n) and imaginary
(k) part of the refractive index of the Nd-doped
polymer waveguide layer can be calculated and
they are shown in Fig. 5(b). Also shown is the
measured refractive index of an undoped reference
Fig. 4. Photoluminescence spectra of Ls.Nd (10ÿ6 M) and
Bz.Nd (10ÿ2 M) in DMSO-d6 solutions. The excitation wave-
polymer. Outside the resonance region, the re-
length is 515 nm at a pump power of 60 mW.
fractive index of the Nd-doped waveguide layer is
L.H. Sloo et al. / Optical Materials 14 (2000) 101±107
Fig. 6. Optical loss spectrum of an undoped partially ¯uori-
nated polycarbonate waveguide, also shown is the measurement
Fig. 5. (a) Measured and calculated ellipsometric parameter D
as a function of wavelength for a 3 wt% Ls.Nd-doped partially
these polycarbonate waveguides are ideally suited
¯uorinated polycarbonate waveguide. (b) Real (n) and imagi-
for planar waveguide applications.
nary (k) part of the refractive index derived from the simulation
Fig. 7 shows the PL intensity of a 3 wt%. Ls.Nd
data indicated by the solid line in (a). The real index for an
doped ¯uorinated polycarbonate waveguide after
undoped reference waveguide is shown for reference (dashed
line in (b)).
excitation at a wavelength of 515 nm. The dashed
line shows the luminescence measured after exci-
tation and collection of the light from the top of
very similar to that of the undoped layer, indi-
the sample
P 40 mW. The 890 and 1060 nm
cating that the spincoating technique leads to Nd-
luminescence of the Nd3 ion are clearly seen.
doped waveguide layers with similar density as
pure waveguide layers. The maximum value of k is
0.027 at 580 nm which corresponds to an absorp-
tion cross-section of 4:5 10ÿ16 cm2, which is
roughly 2±3 times higher than the literature value
for Rhodamine-B [14]. It is clearly seen that the
high absorption of the lissamine causes a change in
the real part of the refractive index around 580 nm
as described by Kramers±Kronig theory.
Optical loss measurements were performed on
an undoped partially ¯uorinated polycarbonate
waveguide using the prism coupling technique.
The result is shown in Fig. 6. The peak around
1650 nm is due to overtone absorption by C±H
bonds. The band around 1400 nm is attributed to
Fig. 7. Photoluminescence spectra of a 3 wt% Ls.Nd-doped
absorption by C±H bonds and O±H bonds in the
polymer waveguide. The dashed line indicates the spectrum
polymer. The two peaks around 1150 nm arise
collected from the top of the waveguide after excitation at 515
from second overtone absorption by aromatic and
nm at a power of 40 mW. The solid line indicates the spectrum
aliphatic C±H bonds. The background loss at the
after excitation at 515 nm at a power of 140 mW and collected
from the output face of the waveguide using a multimode op-
Nd3 emission wavelengths is <0.05 dB/cm at 1060
tical ®ber. The inset shows the time dependence of the 1060 nm
nm and 0.08 dB/cm at 1305 nm. This indicates that
luminescence upon pulsed excitation.
L.H. Sloo et al. / Optical Materials 14 (2000) 101±107
Spectra taken in the near-infrared region (not
A. According to Dexter [21], this means
shown) also show the 1340 nm luminescence. The
that 3% of the maximum possible energy transfer
emission observed in the lower wavelength region
rate is reached. This could be improved by re-
is due to luminescence of the lissamine, which has
ducing the distance between the lissamine and the
a peak emission wavelength (S1 ! S0 transition)
Nd3 ion by changing the con®guration of at-
at 580 nm. The small peaks at the shoulder of the
tachment of the lissamine. Another possibility to
lissamine luminescence are attributed to an inter-
increase the energy transfer rate is to improve the
ference eect between the waveguide layers. The
spectral overlap between the sensitizer and the
solid line in Fig. 7 is the spectrum observed for
Nd3 ion [22]. In the present case, the energy of
excitation from the top and collecting from the
the triplet state of lissamine matches with the 14 600
output facet of the waveguide
P 140 mW;
cmÿ1 band of Nd3 which shows weak absorption.
spot 5 15 mm2. Again the 890 and 1060 nm
If a sensitizer could be used that matches the
luminescence are observed, but here the lumines-
strong 13 600 cmÿ1 absorption band, the energy
cence of the lissamine is strongly decreased, which
transfer rate could be increased.
is attributed to re-absorption by the lissamine it-
Finally, photo-degradation measurements of
self. This indicates that self-absorption should be
the 580 nm lissamine luminescence were performed
taken into account when designing waveguide
on polymer waveguides doped with 3 wt% (com-
plex) and are shown in Fig. 8(a) for normal inci-
The inset of Fig. 7 shows the time dependence
dence irradiation at three dierent pump powers.
of the 1060 nm PL signal after switching the 515
The luminescence signal was detected normal to
nm pump on and o. An exponential ®t through
the waveguide. For a pump power of 4 mW the
the decay part results in a PL lifetime of 0.8 ls.
luminescence intensity slowly rises to a maximum
This is signi®cantly lower than the decay measured
and then decreases again. At higher pump powers
for complexes in DMSO-d6 solution (2.2 ls),
the same eect is observed, but both the increase
which indicates that quenching by C±H and O±H
and the decrease processes occur faster. The pho-
groups in the polymer matrix also contributes to
to-degradation process may be the result of oxygen
the radiationless deactivation of the Nd3.
induced quenching. Another possibility is that
The energy transfer from the lissamine to the
upon photo-excitation, radicals are formed which
Nd3 ion occurs via the triplet state of the lissa-
react with the sensitizer [23]. Further research is
mine. However, the triplet state can also be
quenched by e.g. O2, that is, always present in
solution or in a polymer ®lm. In order to investi-
gate this, we measured the Nd3 luminescence in-
tensity of Ls.Nd in DMSO-d6 solutions before and
after degassing (not shown). The Nd3 PL inten-
sities were similar, indicating that quenching by O2
does not play a role in the DMSO-d6 solutions. As
it is known that the quenching rate by O2 is about
107 sÿ1, it can be estimated that the intramolecular
energy transfer rate to the Nd3 ion has to be >107
sÿ1. This transfer rate is of the same order of
magnitude as for triphenylene sensitized Eu3 in
acetonitrile solutions [20]. A space ®lling model of
Ls.Nd, in which all atoms are represented by their
Fig. 8. (a) 580 nm lissamine photoluminescence of a 3 wt%
van der Waals radius, shows that the distance
Ls.Nd-doped waveguide at pump powers of 4, 20 and 80 mW.
between lissamine and the Nd3 ion is 7±8
(b) Similar measurements on a 3 and 1 wt% Ls.Nd-doped
waveguide at a pump power of 10 mW. As can be seen, the 1
whereas the eective average Bohr radius for the
wt% luminescence does not show the initial increase in the in-
excited lissamine and the unexcited Nd3 ion is
L.H. Sloo et al. / Optical Materials 14 (2000) 101±107
going on to determine the exact nature of the
coating the polymer waveguides and Hans Lamers
(Akzo Nobel) for performing the prism coupling
Room-temperature photoluminescence of Nd3
complexes in DMSO-d6 solution and partially
[1] S. Hufner, Optical Spectra of Transparent Rare-Earth
¯uorinated polycarbonate waveguides has been
Compounds, Academic Press, New York, 1978.
[2] W.J. Miniscalco, J. Lightwave Technol. 9 (1991) 234.
observed for both direct excitation of the Nd3 ion
[3] E. Desurvire, Sci. Am. 266 (1992) 96.
into a higher excited state, and excitation via a
[4] A.M. Glass, Phys. Today 46 (1993) 20.
highly absorbing sensitizer (lissamine). It is shown
[5] E. Desurvire, Phys. Today 47 (1994) 20.
that the excitation eciency upon excitation via
[6] A. Polman, J. Appl. Phys. 82 (1997) 1.
the sensitizer is 104 times higher than for direct
[7] P.G. Kik, A. Polman, MRS Bull. 23 (1998) 48.
[8] R.T. Chen, M. Lee, S. Nataranja, Z.Z. Ho, D. Robinson,
excitation. The intramolecular transfer rate from
IEEE Photon. Technol. Lett. 5 (1993) 1328.
the sensitizer to the Nd3 ion is >107 sÿ1. The lu-
[9] S. Lin, J. Feuerstein, A.R. Mickelson, J. Appl. Phys. 79
minescence lifetime is relatively short, both in
(1996) 2868.
DMSO-d6 (2.2 ls) and in the partially ¯uorinated
[10] B. Booth, in: L.A. Hornak (Ed.), Polymers for Lightwave
polycarbonate waveguide (0.8 ls), which is at-
and Integrated Optics, Marcel Dekker, New York, 1992.
[11] L.H. Sloo, A. Polman, M.P. Oude Wolbers, F.C.J.M. van
tributed to coupling to O±H and C±H vibrations
Veggel, D.N. Reinhoudt, J.W. Hofstraat, J. Appl. Phys. 83
of the complex and the host material. Good
quality optical waveguides can be spincoated with
[12] G. Stein, E. Wurzberg, J. Chem. Phys. 62 (1975) 208.
optical losses for the undoped polycarbonate
[13] V.L. Ermolaev, E.B. Sveshnikova, Russ. Chem. Rev. 63
waveguide of <0.05 dB/cm at 1060 nm and 0.08
[14] M.P. Wolbers, F.C.J.M. van Veggel, D.N. Reinhoudt, J.
dB/cm at 1340 nm. Strong photo-degradation is
Am. Chem. Soc. 119 (1997) 138.
observed that might be due to the presence of
[15] S.I. Klink, G.A. Hebbink, L. Grave, F.C.J.M. van Veggel,
oxygen in the polymer ®lm or due to radicals,
D.N. Reinhoudt, J.W. Hofstraat, L.H. Sloo, A. Polman,
which are formed upon photo-excitation.
[16] I.B. Berlman, Handbook of Fluorescence Spectra of
Aromatic Molecules, Academic Press, New York, 1971.
[17] R.H. Woudenberg, T.O. Boonstra, Polymers comprising a
¯uorinaed carbonate moiety, International Patent, depos-
ited September 3, 1998, #WO 9838237.
This work is part of the research program of the
[18] H.P. Weber, F.A. Dunn, W.N. Leibolt, Appl. Opt. 12
foundation for Fundamental Research on Matter
[19] M.J. Weber, Phys. Rev. 171 (1968) 283.
and has been ®nancially supported by the council
[20] E. van der Tol, Ph.D. Thesis, University of Amsterdam,
for Chemical Sciences of the Netherlands Organi-
Amsterdam, 1998.
zation for Scienti®c Research (CW-NWO), STW,
[21] D.L. Dexter, J. Chem. Phys. 21 (1953) 836.
the IOP Electro-optics Program and the SCOOP
[22] M.H.V. Werts, J.W. Hofstraat, F.A.J. Geurts, J.W.
Verhoeven, Chem. Phys. Lett. 276 (1998) 196.
program of the European Union. Benno Hams
[23] R.P. Wayne, Principles and Applications of Photochemis-
(Akzo Nobel) is greatly acknowledged for spin-
try, Oxford University Press, Oxford, 1988.
Source: http://www.erbium.nl/wp-content/uploads/2016/08/Optical_properties_of_lissamine_functionalized_Nd_complexes_in_polymer_waveguides_and_solution_-_Opt_Mater_2000.pdf
J. Adv. Vet. Anim. Res., 1(3): 114-118. OPEN ACCESS Available at- http://bdvets.org/JAVAR ORIGINAL ARTICLE Volume 1 Issue 3 (September 2014) DOI: 10.5455/javar.2014.a16 Efficacy of different therapeutic regimens for acute foot rot in adult sheep Mohammad Moin Ansari1,*, Khadim Hussain Dar2, Hilal Ahmad Tantray3, Mohammad Mansoor Bhat4, Shahid Hussain Dar1 and Mehraj ud-Din Naikoo1 1Department of Veterinary Clinical Service Complex, Faculty of Veterinary Science and Animal Husbandry (FVSc & AH), Sher-e-Kashmir University of Agricultural Science and Technology (SKUAST) - Kashmir, India; 2Division of Surgery and Radiology, FVSc & AH, Shuhama, Srinagar, Kashmir-190006, India; 3Department of Veterinary Clinical Medicine Ethics & Jurisprudence, FVSc & AH, SKUAST-Kashmir, India; 4Division of LPT, FVSc & AH, SKUAST-Kashmir, India. *Corresponding author's e-mail: ABSTRACT
ISSN : 2394-4536 (Print) esearch News Vol. 3 No. 1 & 2 December 2014 A Half Yearly News Letter of Indian Virological Society on Research and Development in the Field of Virology IVS President Desk depend on which avian influenza A virus caused the infection. Low pathogenic avian influenza A virus Avian influenza A virus in Human