Technopress.kaist.ac.kr
Biomaterials and Biomedical Engineering, Vol. 1, No. 1 (2014) 13-25 DOI: http://dx.doi.org/10.12989/bme.2014.1.1.013 13
Precise ultrasonic coating and controlled release of sirolimus
with biodegradable polymers for drug-eluting stent
Yoon Ki Joung, Bu Nam Jang, Jong Hee Kang and Dong Keun Han
Center for Biomaterials, Biomedical Research Institute, Korea Institute of Science and Technology,
Hwarangno 14-gil 5, Seongbuk-gu, Seoul 136-791, Korea
(Received January 1, 2014, Revised January 29, 2014, Accepted January 30, 2014)
Abstract. In the current study, a drug-eluting stent coated with biodegradable polymers and sirolimus
was developed by using an ultrasonic nanocoater and characterized in aspects of surface smoothness
and coating thickness. In addition,
in vitro release profiles of sirolimus by changing top coating layer
with different biodegradable polymers were investigated. Smooth surfaces with variable thickness could
be fabricated by optimizing polymer concentration, flow rate, nozzle-tip distance, gas pressure, various
solvents and ultrasonic power. Smooth surface could be generated by using volatile solvents (acetone,
chloroform, and methylene chloride) or post-treating with solvent vapor. Coating thickness could be
controlled by varying injection volume or polymer concentration, and higher concentration could
reduce the coating time while obtaining the same thickness. The thickness measurement was the most
effectively performed by a conventional cutting method among three different methods that were
investigated in this study. Release profiles of sirolimus were effectively controlled by changing
polymers for top layer. PLGA made the release rate 3 times faster than PDLLA and PLLA and all top
layers prevented burst release at the initial phase of profiles. Our results will provide useful and
informative knowledge for developing drug-eluting stents, especially coated with biodegradable
polymers.
Keywords: biodegradable polymer; drug-eluting stent; ultrasonic coating; sirolimus; controlled release
1. Introduction
Atherosclerosis is the major cause of coronary heart disease, which shows the narrowing of
arterial intima, named stenosis, which has been described by researchers such as Waller and Slack (1996). Kivelä and Hartikainen (2006) reviewed that over the past decade, several kinds of drug-eluting stent (DES), such as Cypher® SelectTM (sirolimus-eluting stent, Johnson & Johnson) and Taxus® (paclitaxel-eluting stent, Boston Scientific), have become a common solution to treat the coronary arterial stenosis. Moses and Leon (2003) and Maisel (2007) described that although DESs decreased significantly the restenosis rate, it leads to late stent-thrombosis (LST), which recent clinical results showed evidences of a significant increase as compared to bare metal stent (BMS). For the DESs, drugs have been coated with non-degradable polymers such as poly(n-butyl methacrylate) (PBMA)/ poly(ethylene vinyl acetate) (PEVA) and poly (styrene-b-isobutylene-b-
Corresponding author, Ph.D., E-mail:
[email protected]
Copyright 2014 Techno-Press, Ltd.
http://www.techno-press.org/?journal=bme&subpage=7 ISSN: 2288-3738 (Print), 2288-3746 (Online)
Yoon Ki Joung, Bu Nam Jang, Jong Hee Kang and Dong Keun Han
styrene) (SIBS) like studies of Kuchulakanti (2006), Choi (2009), and Commandeur (2006). Costa and Simon (2005) proposed that these polymers are one of the causes generating the LST and inflammation after angioplasty because the non-degradable polymers in stent still remain even after complete release of drug. In contrast, it is well-known that biodegradable polyesters such as poly(lactic acid) (PLA), poly(glycolic acid) (PGA), and poly(lactic-co-glycolic acid) (PLGA) are degraded to monomers or oligomers types such as lactic acid and glycolic acid, ultimately carbon dioxide and water through
in vivo hydrolysis of the ester linkage. Eventually, a novel type of DES that is coated with a biodegradable polymer was recently commercialized (BioMatrix FlexTM, Biosensors). Biosensors reported that the novel stent shows successful clinical results, which show confirmed safety (16% reduction in major adverse cardiac events (MACE)), reduced cardiac death rate (50.5%), 10 times better strut coverage, and more than 20 times better stent apposition, as compared to Cypher® SelectTM stent. Nevertheless, it seems to still afford to improve the performance in terms of physical and pharmaceutical properties, which the process conditions have not been still exhibited.
Several coating methods, which contain dip-coating, spray coating, electrophoretic deposition
technique, electro-spinning, electrospray, layer-by-layer (LbL), and ink-jet deposition, have been employed to develop DES, of which coating thickness and surface morphology are regulated, by Kim and Lee (2010), Nam (2007), Kuraishi and Iwata (2009), Jewell and Lynn (2008), and Wallace and Hayes (2004). It is also important factor which method is cost-effective due to the faster process of stent coating. Although dip-coating is general method to coat metal surface through simple process, a coating material is infiltrated into a crack in the 3-dimensional structure and result in bridging of the spacing. Peng and Chen (2009) proposed that LbL method can construct very stable multi-ultrathin film in structure regardless of coating thickness and conformation because each layer is connected by electrostatic interaction and hydrogen or covalent bond, described by Wang and Zhang (2008). Electrospray technique can easily create an atomized nanostructure using deformed solution which is sprayed from an ultra-fine nozzle when a high voltage is applied to a sample solution. This method has a merit because an application-related industry is capable of fabricating due to the simple device structure and easy coating process. Therefore, this technology has been widely used due to the inexpensive coating process, ultimately reduces the total cost of devices. Gu and Yi (2008), and Lee and Berger (2006) described that ultrasonic coating is an emerging method for coating. The ultrasonic coating uses high-frequency ultrasound waves that can precisely control the thickness of coating by spraying droplets ranging from nano- to micro-meters. According to Pan and Tang (2007), the drug-loaded PLGA surface created by ultrasonic coater showed no webbing and spacing on stent surface, as well as no cracks of polymer coating were observed after stent expansion.
Controlling surface smoothness and coating thickness are very important in drug coating for
stent. Rough surface and thick layer of DES may induce an injury at the lesion site after stent implantation. Santin and Mikhalovska (2004) reported that inflammatory cells are increased at the injury site, which send biochemical signals related with smooth muscle cell (SMC) proliferation. Controlling surface smoothness has crucible means because an injury induced by rough surface provides a cause of SMC proliferation as well as pro-thrombogenic events. Controlling coating thickness is also a core technology because the thinner coating layer can result in lowering the amount of problematic coating polymer and enhancing both surface and bulk stability of coating matrix, which potentially reduces restenotic and/or thrombotic problems. Coating process like these is closely involved in the controlled elution of drug from stent, which depends on parameters such as coating thickness, drug distribution in matrix, degradation rate, and multi-layer
Precise ultrasonic coating and controlled release of sirolimus with biodegradable polymers
Sirolimus is a natural macrocyclic lactone and known as a potent immunosuppressive agent,
which has been widely used for DES during the last decade, reviewed by Wessely and Hausleiter (2005). According to Sehgal (1998), the pathological mechanism is known that it down-regulates mammalian target of Rapamycin (mTOR), thus induces cell-cycle arrest in the late G1 phase, in which the action is only caused by binding to the sirolimus binding protein (FKBP) expressed on the membrane surface of SMC.
In the current study, a drug-eluting stent coated with biodegradable polymers and sirolimus was
developed and characterized in aspects of surface smoothness and coating thickness. In addition,
the release profiles of sirolimus were investigated
in vitro by varying top coating layer with
different biodegradable polymers.
2. Materials and methods
2.1 Materials
Poly(L-lactide) (PLLA,
M
46,000 and 110,000 g/mol, Boehringer, German), poly
(lactic-co-glycolic acid) (PLGA 50 : 50,
M
40,000 g/mol, Boehringer, German), chloroform
(Sigma-Aldrich, USA), acetonitrile (Sigma-Aldrich, USA), dioxane (Sigma-Aldrich, USA), acetone (Sigma-Aldrich, USA), tetrahydrofuran (THF, J.T. Baker, USA), and methylene chloride (MC, J.T. Baker, USA) were obtained as analytic grade. The metal substrates of stainless steel 316L such as stent (1.8 × 0.17 × 18 mm3, where each figure means outside diameter (OD), strut-thickness, and length, respectively, Abbott Korea) and spring (1.8 × 0.25 × 18 mm3, where each figure means outside and inside diameters (OD, ID), and length, respectively, MICROSPRING, Republic of Korea) were purchased. Sirolimus were received from Romark Laboratories LC (USA).
2.2 Preparation of polymer-coated stents
PLGA solution dissolved in 10 mL of solvent was homogeneously mixed using a sonicator
(Ultrasonic 2010, Jinwoo, Korea) for 6 hrs at room temperature. The metal substrates of stent or spring were immersed in acetone for 1 day to wash the surface completely before coating, and then dried for 1 day at 60 oC after cleaning with ultra-pure water 5 times. The coating of the cleaned substrates was carried out using an ultrasonic nanocoater (SONO-TEK, USA) as shown in Fig. 1. The polymer layers coated on substrates were measured with field emission scanning electron microscopy (FE-SEM, Hitachi S-25000, 15 kV, Japan). We evaluated both the surface morphology and thickness of coating using FE-SEM after depositing a thin platinum layer using an ion sputtering system (E-130, 0.1 × 0.05 torr, 10 mA, 80 sec).
2.3 Optimization of coating conditions
2.3.1 Coating solvent PLGA was dissolved in different solvents containing acetone, chloroform, THF, acetonitrile,
dioxane, and MC to give a 0.1 wt% solution and subsequently sprayed on substrates under following coating conditions; flow rate of 0.05 mL/min, eluting amount of PLGA solution of 0.5
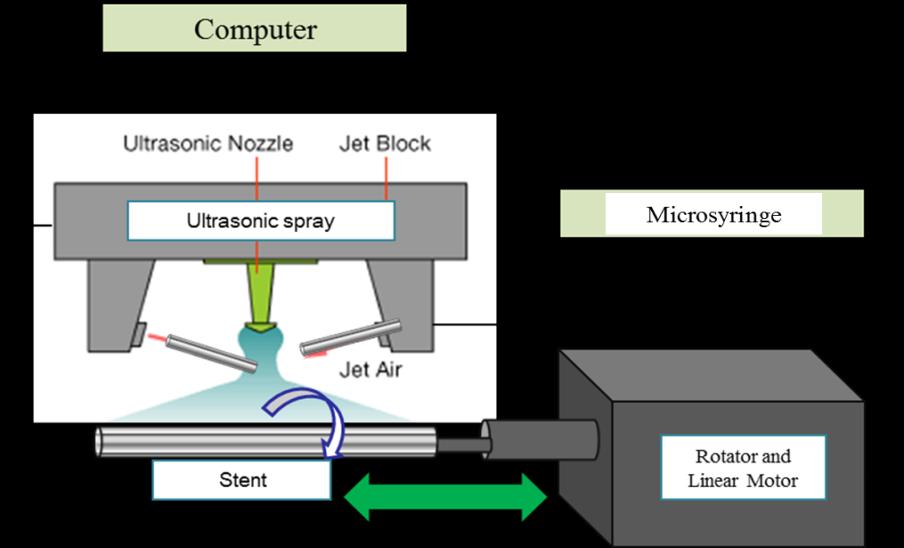
Yoon Ki Joung, Bu Nam Jang, Jong Hee Kang and Dong Keun Han
Fig. 1 Schematic illustration of ultrasonic spray coating method
Table 1 Coating conditions varying the thickness of coating layer
Polymer concentration
Injection volume
mL, spay distance between substrate and nozzle tip of 9 mm, rotating velocity of mandrel of 100 rev./min, and gas pressure of 5 psi. The coating was performed for 20 min by separately coating 2 times at a relative humidity of 40 50% and room temperature.
2.3.2 Polymer concentration PLGA solutions with different concentrations of 0.1, 0.2, 0.3, 0.5 and 0.7 wt% were prepared in
chloroform, and subsequently sprayed on substrates according to different flow rates and eluting-amount under following coating conditions; spay distance of 9 mm, rotating velocity of 100 300 rev/min, and gas pressure of 5 psi. The detailed conditions for flow rate and eluting amount were presented in Table 1. The coating was performed by half coating 2 times at a relative humidity of 40 50% and room temperature. We optimized the most rapid coating time showing a smooth surface and an average thickness ranging from 5 to 6 μm.
2.3.3 Elution volume PLGA solutions with concentration of 0.2 wt% were made in chloroform, and subsequently
sprayed on substrates at different elution volumes of 0.2 1.0 mL under following coating conditions; flow rate of 0.035 mL/min, spay distance of 9 mm, rotating velocity of 300 rev./min, ultrasonic power of 0.75 W and gas pressure of 5 psi. The coating was performed by half coating 2 times at a relative humidity of 40 50% and room temperature.
Precise ultrasonic coating and controlled release of sirolimus with biodegradable polymers
2.4 Surface smoothening by solvent evaporation treatment PLGA solutions (0.1 wt%) were sprayed on stent under following coating conditions; flow rate
of 0.05 mL/min, eluting amount of 0.5 mL, spay distance of 9 mm, rotating velocity of 300 rev./min, ultrasonic power of 0.75 W and gas pressure of 5 psi. The coated stents were hanged on the rack not contacted with the solvent surface in a 110-mL vial containing THF of 40 mL. The vial was maintained at 30 C and the distance between rack and solvent surface was kept at 350 mm. Surface morphology was observed at three vaporization times of 0, 20 and 90 sec with FE-SEM.
2.5 Measurement of coating thickness
Three different methods were performed to investigate which method is accurate to measure the
thickness of coating layers of stent; (1) cutting method, (2) cold mounting method, and (3) focused ion beam (FIB) Ga milling method. In cutting method, sample was cut with a diamond scissor after experiencing quick chilling by soaking it into liquid nitrogen, and then its cross section was evaluated with FE-SEM. For cold mounting, we made hardening resin solution for treating the sample with cold mounting. Epoxy-hardening resin and rapid-hardening solution (hardener) were mixed and then the mixed solution left undone for 10 min at room temperature for inhibiting minimum gas bubbles from rising. Subsequently, the solution was inserted into mold with a specific scale after inserting the solution into syringe. It is required to cool the mold because a mild heat generates when mixing the hardening resin and the solution. Immersing the mold into ice water bath enables to hinder the polymers from exceeding the scope of glass temperature. Polishing of the hardening resin using sandpaper (200 2,400) and suspension was performed after drying at room temperature under vacuum for 1 day. Finally, the cross-section of the sample was observed with both FE-SEM and optical microscopy (OM, EL-Einsatz, Carl Zeiss, German). For FIB Ga milling, the minimum step for decreasing the heat damage of polymer was taken after depositing the fine focused Ga2+ ions on the sample pre-treated with Pt-Pb, and then the measurement of polymer thickness was performed by focused ion beam-SEM (FIB-SEM, Helios 600 D456, FEI Co., Netherlands).
2.6 Release test Sirolimus-coated springs were immersed in a 10-mL vial containing 1 mL PBS solution (pH
7.4). The vials were incubated in a shaking water bath at 37 C and 100 rpm. At fixed time intervals, the incubated PBS was removed for the measurement of released sirolimus. The amount of sirolimus extracted to a mixed solution (DCM:PBS = 1:1, v/v) was determined by high performance liquid chromatography (HPLC, LC1100, Agilent, USA) with a C18 reverse-phase column (Eclipse XDB-C18, 4.6 mm 150 mm, 5 μm). The mobile phase was methanol/0.5 mM ammonium acetate (85:15, v/v). The flow rate was 1 mL/min and the UV absorbance was recorded at 245 nm.
3. Results and discussion
The aim of this study was to investigate conditions of coating process to achieve smooth
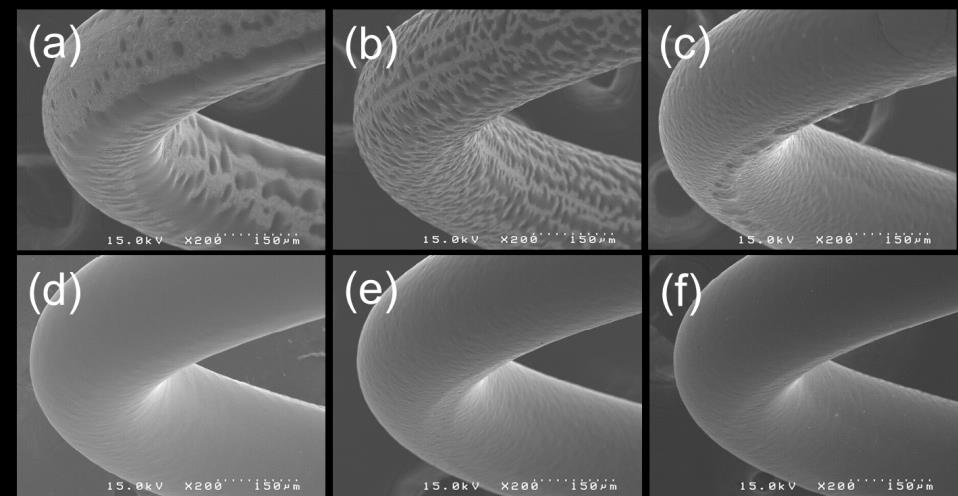
Yoon Ki Joung, Bu Nam Jang, Jong Hee Kang and Dong Keun Han
coating surface and adjustable thickness during an effective spray time by varying coating solvent, flow rate, polymer concentration, elution volume, and ultrasonic power. Additionally, based on these optimized coating conditions, it was investigated how the release profiles of sirolimus can be controlled by varying polymer to subsequently coat on the coated layer as an additional top layer.
Table 1 summarizes the variation of thickness at different coating conditions. When the
concentration of PLGA solutions was changed from 0.1 to 0.7wt%, the elution volumes were decreased to adjust the amount of polymer to be similar. Thus, the coating time was decreased in accordance with elution volume. The resulting thickness was varied ranging from 5.4 to 6.2 μm, which the tendency of thickness changes is not correlated with any coating conditions. This means that the thickness variation can be affected by not single condition but various conditions even though the same amount of polymer to spray for coating provides similar thicknesses with differences of below 0.8 μm. Solvent for polymer coating is very important for controlling surface morphology as well as other properties such as release profile or degradation rate. Fig. 2 shows Scanning electron microscopic images of springs coated with PLGA solutions that were dissolved in various solvents. Dioxane and acetonitrile (Fig. 2(a) and (b)) show distinctly rougher surfaces as compared with other solvents. THF (Fig. 2(c)) is slightly rough and acetone, chloroform, and methylene chloride made comparatively smooth surface. On the assumption that the solubility of PLGA for all solvents is the same, surface morphology can be explained by physical properties of solvents. Three solvents that made smooth surfaces are largely more volatile than others, which the tendency is evidently shown in terms of volatility and boiling point. Dioxane and acetonitrile that
show very rough surfaces has boiling points of 101 and 82 C, respectively, whereas acetone and
methylene chloride that made smooth surfaces those of 56 and 40 C, respectively. Based on the fact that solvents having lower boiling points are more volatile, it is concluded that volatile solvents make smooth surfaces during ultrasonic coating process.
Coating thickness can be easily controlled by varying feed amount of polymer solution. Fig. 3
shows that the thickness increases in definite proportion to injection volume. This indicates that a precise control of coating thickness is feasible by the regulation of injection volume. As shown in Figs. 3B(a) 3B(e), increasing thickness in cross-sections was clearly observed as increasing the injection volume. It is readily predicted that larger volume of injection results in thicker coating
Fig. 2 SEM images of stainless steel (SS) springs coated with PLGA solution dissolved in different solvents;
(a) dioxane, (b) acetonitrile, (c) THF, (d) acetone, (e) chloroform and (f) methylene chloride

Precise ultrasonic coating and controlled release of sirolimus with biodegradable polymers
A. Thickness variation versus injection volume
B. SEM images of the cross-section of coating layer
Fig. 3 Thickness variation of springs coated with PLGA solution at different injection volumes; (a) 0.2, (b)
0.4, (c) 0.6, (d) 0.8 and (e) 1.0 mL
layer of polymer on stent surface under identically maintained other conditions. However, thickening the coating layer for increasing drug amount may cause other problems when implanted in vivo, such as surface cracks due to lowered elasticity, deteriorated inflammatory response or late/very late thrombosis, and uncontrolled release profile of drug. Therefore, the injection volume should be regulated by considering other conditions, such as polymer concentration and drug amount in solution, to obtain the desired thickness.
Although the thickness of a coated polymer on metallic surfaces can be measured by various
methods, the accuracy depends on what method is adequate to measure that. Fig. 3 shows SEM images of cross-sections generated by three different cutting methods. Cutting method (Fig. 4(a)) can easily measure a coating thickness and has the advantage that the measurement time is short. However, the reliability of a measured thickness is low because a local force is transferred at the surface when cutting a coating stent, leading to destruction and deformation. FIB Ga2+ milling method (Fig. 4(b)) measures an etched thickness by removing of a little amount through sputtering at high primary currents. Relatively longer time is required for the measurement although this is simple method. The accuracy of thickness measurement is a little lower than that of cutting method because heat damage does not decrease after deposition. On the other hand, cold mounting method (Fig. 4(c) had the highest accuracy of thickness measurement among three methods. That is explained that the coated substrate is well-preserved because both physical force and heat damage from external region are not generated. In this study, cutting method was used because it can be performed at simple process and has proper accuracy in thickness measurement.
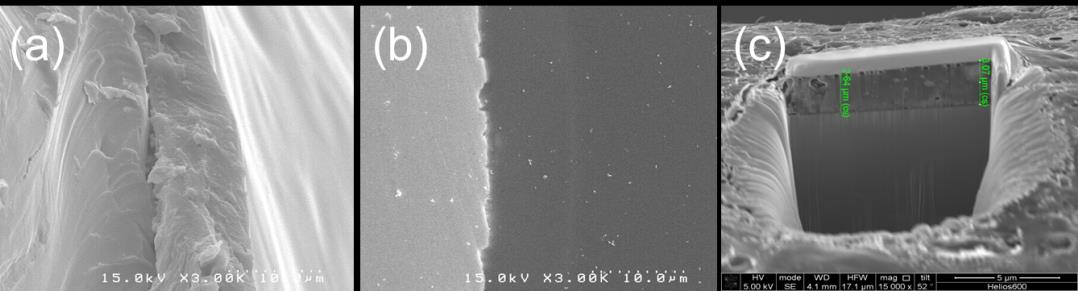
Yoon Ki Joung, Bu Nam Jang, Jong Hee Kang and Dong Keun Han
Fig. 4 SEM images showing the cross-section of PLGA-coated stents different cutting methods for thickness
measurement; (a) cutting method, (b) cold mounting method and (c) FIB Ga milling method
Fig. 5 ATR-FTIR spectra of (a) sirolimus, (b) PLGA film, and (c) PLGA film containing 20 wt% sirolimus
Fig. 5 presents FTIR spectra of PLGA layer containing sirolimus along with PLGA layer only
and sirolimus. The spectrum of drug-containing layer contained all characteristic signals of both PLGA and sirolimus as readily estimated. Characteristic signals of sirolimus such as CH, CN,
and C=N were presented at the wavelengths of around 2950, 1634, and 1645 cm 1, respectively. Additionally, it is also confirmed that there was no unexpected chemical reactions due to the absence of new signal that does not observed in both materials.
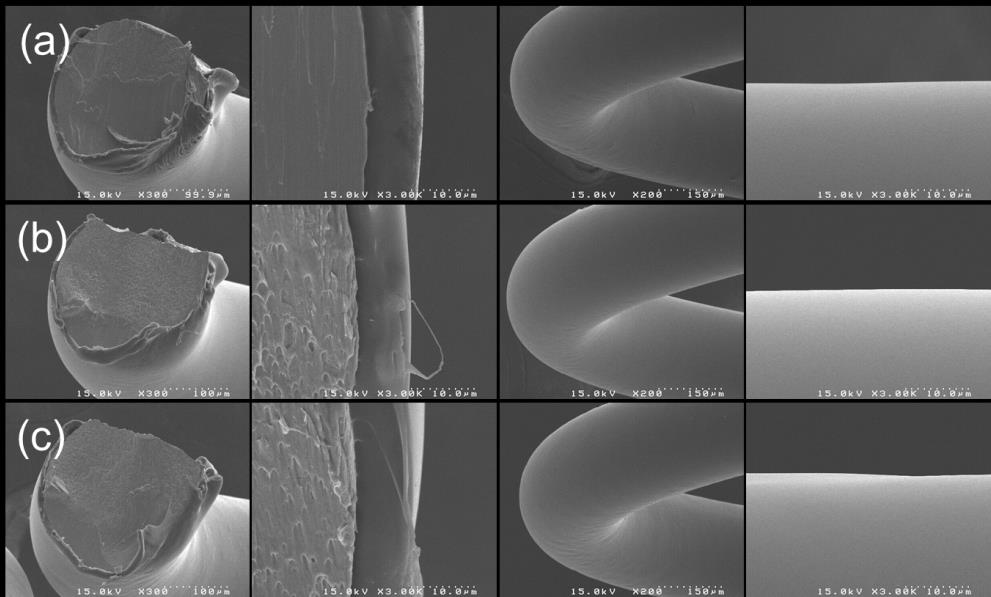
Precise ultrasonic coating and controlled release of sirolimus with biodegradable polymers
Fig. 6 SEM images showing the cross-section of spring coated with PLGA (50:50, 40K) containing sirolimus
and additionally coated as a top layer with different polymers; (a) PDLLA (41K), (b) PLGA (50:50-40K) and (c) PLLA (46K)
After optimizing coating process, release profiles of sirolimus were investigated with the
variation of polymer for a top layer. Fig. 6 shows microscopic images of the cross-section of spring generated by cutting method, in which the spring was coated with PLGA containing sirolimus and subsequently coated with PDLLA, PLGA, or PLLA that did not contain any drug. Although distinct lines cannot be drawn between top and bottom layers, polymers were well coated and generated smooth surfaces. This also shows that well-mixed two layers that were not distinguished possibly are favourable for coating stability and drug release of sirolimus-eluting stent.
Fig. 7 presents release profiles of sirolimus from spring coated with different polymers for top
layer. The release rate of PLGA is much faster than those of PDLLA and PLLA due to the fast degradation rate. The cumulative release of PLGA was almost 100% at 28 days after incubation. For PDLLA and PLLA, the release rates were more than 3 times lower than that of PLGA, which the cumulative release was about 1530% at 28 days. PDLLA has a little faster degradation rate than PLLA and the release profiles are in accordance with the degradation rate. More importantly, all profiles did not present any burst release at initial phase of incubation period, as compared to the case without top layer (data not shown).
For smoothening of a rough surface of polymer-coated stent, additional process was
investigated. Fig. 8 shows SEM images of PLGA-coated stent surfaces treated by THF vaporization for 0, 20 and 90 sec. The surfaces of PLGA-coated stent that coated with some solvents (Figs. 2ac) have been severely or slightly rough. After 20-sec THF treatment, the surface became much smoother than that before the treatment (Fig. 8a). However, in the case of the treatment for 90 sec (Fig. 8c), webbings between struts were observed (yellow circle). This probably was because coated PLGA on strut was excessively dissolved by THF vapour for long treatment time. Thus, it is important that adequate treatment time of solvent vapour can make a rough surface to a smooth surface. Problems like webbing may be critical when a DES is implanted in vivo. This is because the excessive amount of synthetic polymers is possibly not favourable for inhibiting in-stent inflammation and late thrombosis. Post-treatment with solvent
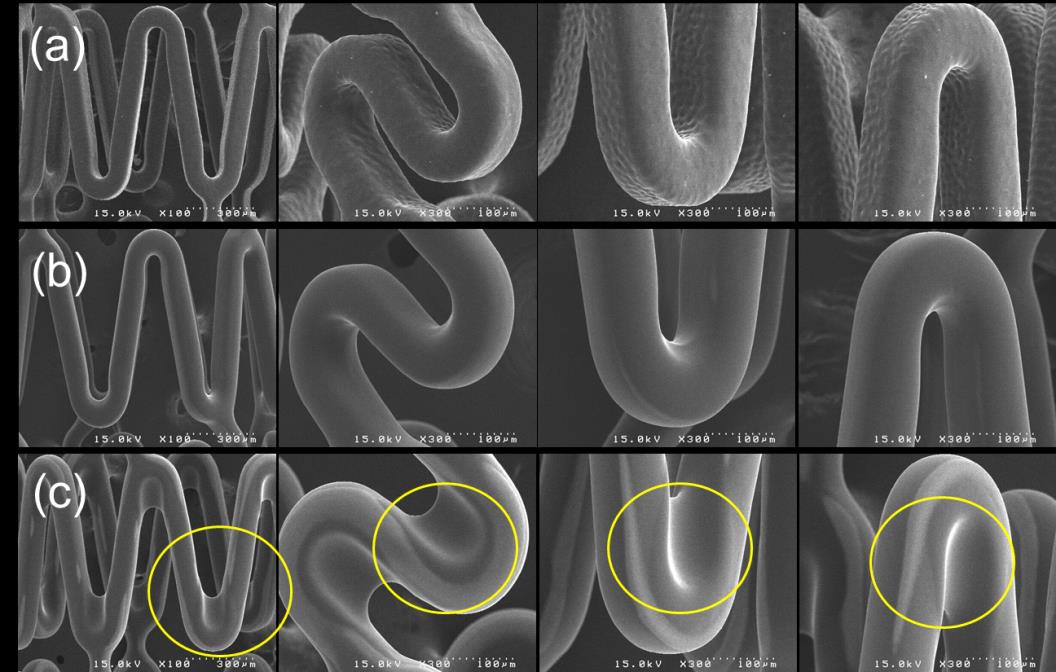
Yoon Ki Joung, Bu Nam Jang, Jong Hee Kang and Dong Keun Han
vapour can be more beneficial than heat treatment. Solvent vapour probably mainly deforms the surface morphology of polymeric coating layer rather than the bulk microstructure. Heat treatment changes bulk microstructures, thermal degradation, and rearrangement of polymer chains. The rearrangement of polymer chains will presumably cause deteriorated physical and pharmacokinetic properties.
Fig. 7 In vitro cumulative release profiles of sirolimus from stents coated with PLGA (50:50, 40K) and
additionally coated with different polymers at a top layer: (a) PDLLA (41K), (b) PLGA (50:50-40K) and (c) PLLA(46K)
Fig. 8 SEM images of the PLGA-coated stents treated with different times of THF vaporization: (a) 0, (b) 20
Precise ultrasonic coating and controlled release of sirolimus with biodegradable polymers
Neointimal hyperplasia resulting in stent stenosis remains the major concern after the stent
implantation. Although, in recent randomized clinical trial, the restenosis rate after the DES placement is lower than both a conventional balloon angioplasty and intervention with a BMS, this beneficial effect of DES was not related to the inhibition of the neointimal cellular proliferation following vascular injury but simply to the mechanical result of overstretching the treated vessel segment, which was described by Kuntz and Gibson (1993) and Stone and Ellis (2004). Generally, DES becomes rigid as compared to BMS after coating because coating polymers decrease inherent flexible property of BMS strut. The pathogenesis of neointimal hyperplasia (restenosis) and thrombosis after coronary interventions and stent implantation is not well understood. However, it is known that major reasons seem to be 1) a healing response due to the vessel injury caused by balloon dilatation and stent implantation, 2) platelet activation resulting in the release of coagulating and signaling factors, 3) thrombus formation resulting in thrombus organization, and 4) foreign body response to the stent resulting in an inflammatory response. To improve currently used coronary DESs, researchers will have to focus on these factors. The impact of the various factors on these problems is not well understood. However, we believe that these factors may be partially regulated by precise control of coating properties and release profiles.
The surface morphology of DES plays an important role in clinical stent implantation. That is, a
DES must be fabricated to have a smooth surface without webbings between stent struts. Smooth
surface coating can not only significantly decrease the injury of blood vessel but also make the
stent go to injury site successfully. Scheerder and Wilczek (1995) reported that the smooth surface
of stent struts causes less thrombus formation after insertion as compared to the stent with rough
surface. In addition to the smooth and uniform surface, the polymer coatings for drug delivery
from stents also need to satisfy a legion of physical, biological, and regulatory criteria. Scheerder
and Wang (1996) mentioned that the stent coating should withstand subsequent processing, such as
sterilization, mount, and deployment without compromising the physical integrity of the coating.
4. Conclusions
In conclusion, a drug-eluting stent coated with biodegradable polymers, at top and bottom layer,
and sirolimus was well fabricated by using an ultrasonic nanocoater and optimized in terms of
surface smoothness and coating thickness. Additionally, in vitro release profiles of sirolimus were
effectively controlled by changing polymers for top layer. Therefore, all of results will contribute
to develop emerging DES coated with biodegradable polymers exhibiting advanced efficacy and
safety.
Acknowledgments
This work was supported by Pioneer Research Center Program (2012-0001047) and Cell
Regeneration Program (2012M3A9C6049717) through the National Research Foundation of Korea
funded by the Ministry of Science, ICT & Future Planning (MSIP), Industrial Strategic Technology
Development Program (10043971) funded by Ministry of Trade, Industry and Energy (MOTIE),
Republic of Korea.
References
Yoon Ki Joung, Bu Nam Jang, Jong Hee Kang and Dong Keun Han
Bennett, M.R. and O'Sullivan, M. (2001), "Mechanisms of angioplasty and stent restenosis: implications for
design of rational therapy", Phamacol. Ther., 91(2), 149-166.
Choi, J., Konno, T., Takai, M. and Ishihara, K. (2009), "Controlled drug release from multilayered
phospholipid polymer hydrogel on titanium alloy surface", Biomaterials, 88(28), 5201-5208.
Commandeur, S. and Van Beusekom H.M.M. (2006), "Polymers, drug release, and drug-eluting stents", J.
Intervent. Cardiol., 19(6), 500-506.
Costa, M.A. and Simon, D.I. (2005), "Molecular basis of restenosis and drug-eluting stents", Circulation,
111, 2257-2273.
De Scheerder, I.K., Wang, K. and Wilczek, K. (1996), "Local methylprednisolone inhibition of foreign body
response to coated intracoronary stents", Coron. Artery. Dis., 7(2),161-166.
De Scheerder, I.K., Wilczek, K. and Verbeke, E.V. (1995), "Biocompatibility of polymer-coated oversized
metallic stents implanted in normal porcine coronary arteries", Atherosclerosis, 1(14), 105-114.
Gu, X.Z., Yi, H., Ni, Z.H. and Fang, J.H. (2008), "Drug-eluting coronary stents coated with
polysulfone-poly(ethylene oxide) block copolymer by ultrasonic spray", Key Eng. Mater., 373-374,
633-636.
Jewell, C.M. and Lynn, D.M. (2008), "Multilayered polyelectrolyte assemblies as platforms for the delivery
of DNA and other nucleic acid-based therapeutics", Adv. Drug Deliv. Rev., 60(9-10), 979-999.
Kim, D.M., Lee, B.S., Park, C.H., Park, K., Son, T.I., Jeong, M.H. and Han, D.K. (2010), "Metal surface
coating using electrospray of biodegradable polymers and behavior of ALA release for drug-eluting stents",
Polym. Korea, 34(2), 172-177.
Kivelä, A. and Hartikainen, J. (2006), "Restenosis related to percutaneous coronary intervention has been
solved?", Ann. Med., 38(3), 173-187.
Kuchulakanti, P.K., Chu, W.W., Torguson, R., Ohlmann, P., Rha, S.W., Clavijo, L.C., Kim, S.W., Bui, A.,
Gevorkian, N., Xue, Z., Smith, K., Fournadjieva, J., Suddath, W.O., Satler, L., Pichard, F.A., Kent, D.K.M.
and Waksman, R. (2006), "Correlates and long-term outcomes of angiographically proven stent
thrombosis with sirolimus- and paclitaxel-eluting stents", Circulation, 113, 1108-1113.
Kuntz, R.E., Gibson, C.M. and Nobuyoshi, M. (1993), "Generalized model of restenosis after conventional
balloon angioplasty and new devices", J. Am. Coll. Cardiol., 21(1), 15-25.
Kuraishi, K., Iwata, H., Nakano, S., Kubota, S., Tonami, H. and Toda, M. (2009), "Development of
nanofiber-covered stents using electrospinning: in vitro and acute phase in vivo experiments", J. Biomed.
Mater. Res. B. Appl. Biomater., 88b(1), 230-239.
Lee, L.Y. and Berger, H.L. (2006), "Coating drug-eluting arterial stents using ultrasonic spray nozzles",
Proceedings of the 19th annual conference on liquid atomization and spray systems, Toronto, Canada, May.
Maisel, W.H. (2007), "Unanswered questions-drug-eluting stents and the risk of late thrombosis", N. Engl. J.
Med. 365, 981-984.
Moses, J.W., Leon, M.B., Popma, J.J., Fitzgerald, P.J., Holmes, D.R., O'Shaughnessy, C., Caputo, R.P.,
Kereiakes, D.J., Williams, D.O., Teirstein, P.S., Jaeger, J.L. and Kuntz, R.E. (2003), "Sirolimus-eluting
stents versus standard stents in patients with stenosis in a native coronary artery", N. Engl. J. Med., 349,
1315-1323.
Nam, S.H., Nam, H.Y., Joo, J.R., Baek, I.S. and Park, J.S. (2007), "Curcumin-loaded PLGA nanoparticles
coating onto metal stent by electrophoretic deposition techniques", Bull. Korean Chem. Soc., 28(3),
397-402.
Nebeker, J.R., Virmani, R., Bennett, C.L., Hoffman, M., Samore, J.M.H., Alvarez, J.C., Davidson, J., McKoy,
J.M., Raisch, D.W., Whisenant, B.K., Yarnold, P.R., Belknap, S.M., West, D.P., Gage, J.E., Morse, R.E.,
Gligoric, G., Davidson, L. and Feldmanet, M.D. (2006), "Hypersensitivity cases associated with
drug-eluting coronary stents: a review of available cases from the research on Adverse Drug Events and
Reports (RADAR) project", J. Am. Coll. Cardiol., 47(1), 175-181.
Pan, C.J., Tang, J.J. and Weng, Y.J. (2007), "Preparation and characterization of rapamycin-loaded PLGA
coating stent", J. Mater. Sci. Mater. Med., 18(11), 2193-2198.
Peng, H.Y., Chen, M., Zheng, B., Wang, X.G. and Huo, Y. (2009), "Long-term effects of novel biodegradable,
Precise ultrasonic coating and controlled release of sirolimus with biodegradable polymers
polymer-coated, sirolimus-eluting stents on neointimal formation in a porcine coronary model", Int. Heart
J., 50(6), 811-822.
Santin, M., Mikhalovska, L., Lloyd, A.W., Mikhalovsky, S., Sigfrid, L., Denyer, S.P., Filed, S. and Teer, D.
(2004), "In vitro host response assessment of biomaterials for cardiovascular stent manufacture", J. Mater.
Sci. Mater. Med., 15(4), 473-477.
Sehgal, S.N. (1998), "Rapamune® (RAPA, rapamycin, sirolimus): mechanism of action immunosuppressive
effect results from blockade of signal transduction and inhibition of cell cycle progression", Clin.
Biochem., 31(5), 335-340.
Stone, G.W., Ellis, S.G., Cox, D.A., Hermiller, J., O'Shaughnessy, C., Mann, J.T., Turco, M., Caputo, R.,
Bergin, P., Greenberg, J., Popma, J.J. and Russell, M.E., (2004), "A polymer-based, paclitaxel-eluting stent
in patients with coronary artery disease", N. Engl. J. Med., 350, 221-231.
Wallace, D., Hayes, D. and Chen, T. (2004), "Ink-jet deposition of materials for MEMS packaging",
Proceedings of the 6th topical workshop on packaging of MEMS and related micro-nano-bio integrated systems, Long Beach, California, November.
Waller, B.F., Fry, E.T.A., Hermiller, J.B., Peters, T. and Slack, J.D. (1996), "Nonatherosclerotic causes of
coronary artery narrowing-Part I", Clin. Cardiol., 19(6), 509-512.
Wang, X., Zhang, X. Castellot, J., Herman, I., Iafrati, M. and Kaplan, D.L. (2008), "Controlled release from
multilayer silk biomaterial coatings to modulate vascular cell responses", Biomaterials, 29(7), 894-903.
Wessely, R., Hausleiter, J., Michaelis, C., Jaschke, B., Vogeser, M., Milz, S., Behnisch, B., Schratzenstaller,
T., Renke-Gluszko, M.,M., Wintermantel, E., Kastrati, A. and A. (2005), "Inhibition of
neointima formation by a novel drug-eluting stent system that allows for dose-adjustable, multiple, and
on-site stent coating", Arterioscler. Thromb. Vasc. Biol., 25(4), 748-753.
Source: http://technopress.kaist.ac.kr/download.php?journal=bme&volume=1&num=1&ordernum=2
Water Dispersible Granular Biofungicide Active Ingredient: Net Weight: 5 Pounds Bacillus amyloliquefaciens strain D747* . . . . . . . . . . . . . 25.0% EPA Reg. No. 70051-108 EPA Est. No. 70051-CA-001 *Contains a minimum of 5×1010 colony-forming units (cfu) per gram Certis USA, L.L.C. KEEP OUT OF REACH OF CHILDREN
Online Submissions: wjg.wjgnet.com World J Gastroenterol 2009 July 7; 15(25): 3073-3085 [email protected] World Journal of Gastroenterology ISSN 1007-9327 doi:10.3748/wjg.15.3073 © 2009 The WJG Press and Baishideng. All rights reserved. EDITORIAL A systematic review of the efficacy and safety of herbal medicines used in the treatment of obesity