Pii: s0013-4686(02)00777-6
Electrochimica Acta 48 (2003) 855 /865
Tris (2,2?-bipyridil) copper (II) chloride complex: a biomimetic
tyrosinase catalyst in the amperometric sensor construction
Maria Del Pilar Taboada Sotomayor a, Auro Atsushi Tanaka b, Lauro Tatsuo Kubota a,
a Instituto de Quı´mica, UNICAMP, P.O. Box 6154, 13083-970 Campinas, SP, Brazil
b Departamento de Quı´mica, UFM, 65070-270 Sa˜o Luı´s, MA, Brazil
Received 25 September 2002; received in revised form 21 November 2002
The use of tris (2,2?-bipyridil) copper (II) chloride complex, [Cu(bipy)3]Cl2×/6H2O, as a biomimetic catalyst, is reported in the
construction of an amperometric sensor for dopamine. The sensor was prepared modifying a glassy carbon electrode with aNafion† membrane doped with the complex. The optimized conditions for the sensor response were obtained in 0.25 mol dm3Pipes buffer (pH 7.0) containing 150 mmol dm3 H2O2, with an applied potential of /50 mV versus saturated calomel electrode(SCE). In these conditions, a linear response range between 9 and 230 mmol dm3, with a sensitivity of 1.439/0.01 nA dm3 mmol1cm2 and a detection limit of 4.8 mmol dm3 were observed for dopamine. The response time for this sensor was about 1 s,presenting the same response for at least 150 successive measurements, with a good repeatability (4.8%) expressed as relativestandard deviation for n /13. After its construction, this sensor can be used after 180 days without loss of sensitivity, kept at roomtemperature. The difference of the sensor response between four preparations was 4.2%. A detailed investigation about the sensorresponse for other eighteen phenolic compounds and five interfering species was performed. The sensor was applied for dopaminedetermination in pharmaceutical preparation with success.
# 2002 Elsevier Science Ltd. All rights reserved.
Keywords: Copper complexes; Biomimetic catalyst; Tyrosinase; Catecholamines; Amperometric sensors
trocatalytic amplification cycle . When the quinoneis reduced quantitatively and reversibly on an electrode
In the last years many biosensors for phenols detec-
surface, the catalytic recycling gives a significant in-
tion have been described in the literature. Most of them
crease in the reduction current that is proportional to
using extracted enzymes from natural sources, purified
the concentration of the phenolic substrate . How-
or lyophilized . The principle of bioelectrocataly-
ever, still exist a necessity to improve the performance of
tic conversion of phenols to quinone by the Tyr
these biosensors, principally due to the short lifetime,
immobilised in these biosensors is well known .
poor stability and relatively high detection limits. These
The phenolic substrate diffuses through the sensitive
problems suggest that the electrochemical reduction step
layer, where it is enzymatically oxidized to quinone
must be improved. An alternative for this is the
species by tyrosinase under the O2 consumption. The
improvement of the catalytic efficiency in the sensitive
substrate is regenerated through the electrochemical
layer. In this sense, Hedenmo et al. demonstrated
reduction of these quinones, which is the critical step
that immobilized an osmium complex (mediator) and
in the response of these biosensor based on tyrosinase
Tyr together, improved the catalytic performance as a
(Tyr). This regeneration leads to a significant amplifica-
consequence of a more efficient mediated electroreduc-
tion of quinone species production, forming a bioelec-
tion, recycling the produced quinones enzymatically.
Another alternative to improve the performance of thesebiosensors for phenols detection is making the electron
* Corresponding author. Tel.: /55-19-3788-3127; fax: /55-19-
transfer easier between the active site of the enzyme and
E-mail address: (L.T. Kubota).
the electrode surface, reducing or removing the protein
0013-4686/02/$ - see front matter # 2002 Elsevier Science Ltd. All rights reserved.
PII: S 0 0 1 3 - 4 6 8 6 ( 0 2 ) 0 0 7 7 7 - 6
M.D.P.T. Sotomayor et al. / Electrochimica Acta 48 (2003) 855 /865
(protective shell) around the active site. In this sense, the
4-Aminophenol, 4-nitrophenol and p -phenylenediamine
use of biomimetic chemistry synthesizing artificially
dihydrochloride were obtained from Riedel, De Hae¨n,
active sites of Tyr , trying to imitate the natural
Germany. Tetrahydrofuran and methanol were acquired
enzyme with the same efficiency and selectivity, is very
from J.T. Baker, Xalostoc, Mexico.
attractive. Based on this context, the term ‘enzymelessbiosensors' can be used For the other hand, the
2.2. Preparation of tris(2,2?-bipyridil) copper(II)
use of biomimetic catalysts will be able to infer more
robustness to the sensors, overcoming the low stabilitypresented by the enzyme.
The complex was synthesized according to the proce-
Various biomimetic catalysts of tyrosinase have been
dure described by Meyer , with some modifications.
described in literature and demonstrating
For 25.0 cm3 of a 0.04 mol dm3 CuCl2×/2H2O aqueous
efficient catalyst to oxidize phenolic and catecholic
solution (1 mmol), was used 25.0 cm3 of a 0.12 mol
substrates to quinones. The redox behavior of all these
dm3 2,2?-bipyridine ethanol solution (3 mmol), and
compounds is very interesting, however, they have rarely
then they were slowly mixed. The solvent in the resulting
been studied in detail, mainly for sensor development.
blue solution was removed under vacuum, until to
With the aim to develop amperometric sensors based on
obtain a volume of 20 cm3. Then, this solution was
biomimetic catalyst of Tyr, an approximation of its
stand at 4 8C, in order to allow slow evaporation of the
active site can be made using molecules containing
remaining solvent, such as recommended by Palmer and
copper atoms surrounded by nitrogen. This approach
Piper After about 30 days, blue color crystals were
mimics the Tyr deoxy form , that is able to
obtained, corresponding to the tris(2,2?-bipyridil) cop-
catalyze the catecholamines oxidation, in the same form
per(II) chloride complex, with formulae [Cu(bipy)3]Cl2×/
that the Tyr. On this context, this work investigates the
6H2O, which was confirmed through elementary analy-
use of tris(2,2?-bipyridil) copper(II) chloride complex as
sis (C, H, N). The obtained data were: 49.0% C; 4.8% H
a biomimetic catalyst of Tyr, in the construction of an
and 11.3% N versus 49.4% C; 4.9% H and 11.5% N, for
amperometric sensor for dopamine determination.
theoretical ones. The Cu amount determined by atomicabsorption spectrometry was 9.1 against 8.7% fortheoretical one.
2.3. Sensor construction
In a first step, solutions containing 1, 2, 3 and 5 g
All chemicals were analytical grade reagent. Buffer
dm3 of the complex in DMF were prepared, in order
and phenol solutions were prepared using de-ionized
to test the influence of the amount of copper (II)
water (Milli-Q Millipore system), and the actual pH of
complex on the sensor construction.
the buffers was determined employing a pH electrode
A glassy carbon (GC) electrode, acquired from
connected to a pH-meter (Corning pH/Ion analyzer 350,
Metrohm†, Switzerland with a geometrical area of
New York, USA).
0.071 cm2, was used for the sensor construction.
Phenol, 30% (w/v) hydrogen peroxide, monopotas-
Initially, the surface of the GC electrode was cleaned
sium phosphate (KH2PO4), sodium hydroxide, sodium
according to the procedure described in the literature
chloride, sodium bisulfite and N,N -dimethyformamide
(DMF) were acquired from Synth, Sa˜o Paulo, Brazil.
After cleaning the GC electrode, 200 mm3 of copper
Catechol, uric acid, 3-nitrophenol, epinephrine, (9/)
complex solution was mixed with 100 mm3 of 5% (w/v)
norepinephrine L-bitartrate hydrate, tetrachloro-1,2-
Nafion† solution, and an aliquot of 150 mm3 of this
benzoquinone, guaiacol and 5% (w/v) Nafion† solution
mixture was put on the GC electrode surface. Finally the
were purchased from Aldrich, Milwaukee, USA. Ascor-
solvent was evaporated at room temperature during 4 h,
bic acid, o -phenylenediamine, 2-aminophenol, 3-amino-
forming a film of 23 mm thickness on the electrode
phenol, 2-nitrophenol and sodium salicylate were from
surface, estimated as described by Langmaier et al.
Merck, Darmstadt-Germany. 3,4-Dihydroxyphenethy-lamine (dopamine), 5-hydroxytryptamine (serotonin),
2.4. Electrochemical measurements
N? -bis[2-ethanesulfonic acid] (Pipes) and N -[2-hydro-
The voltammetric and amperometric measurements
xyethyl]piperazine-N ?-[2-ethanesulfonic acid] (Hepes)
were carried out in a potentiostat from Echo Chemie
were acquired from Sigma, St. Louis, USA. Copper
(Autolab PGSTAT10 model), Utrecht, Netherlands and
(II) chloride dihydrate was acquired from Vetec, Rio de
using an electrochemical cell with three electrodes. A
Janeiro, Brazil. Acetaminophen and 2,2?-bipyridyl
saturated calomel electrode (SCE) was used as reference,
(bipy) were purchased from Acros, New Jersey, USA.
a Pt wire as counter and the GC electrode, modified
M.D.P.T. Sotomayor et al. / Electrochimica Acta 48 (2003) 855 /865
with Nafion† membrane doped with copper (II) com-plex (sensor), as working electrode.
Before the electrochemical measurements the elec-
trode was kept in buffer solution for 20 min, formembrane hydration. The measurements were carriedout in 5.00 cm3 of buffer solution, applying an adequatepotential. Initially the current was continuously mon-itored until it reaches the steady state (from 3 to 5 min).
After that, an addition of hydrogen peroxide solutionwas made into the buffer solution, which it was stirredfor a few seconds (30 s), in order to homogenize thesolution before current monitoring. Then, the currentwas monitored until reach a new steady state. Successiveadditions of phenol standard solutions were performedevery 30 s, always stirring the solution for a few secondsbefore current monitoring.
2.5. Determination of the apparent Michaelis /Mentenconstants (Kapp
The apparent Michaelis
were determined from the electrochemical Eadie /Hofs-tee form of the Michaelis /Menten equation plot-ting Djs/C versus Djs, where js is the current density inthe steady state and C is the concentration of phenoliccompound. In these graphs the Kapp
obtained from their respective slopes. The curves wereplotted considering concentrations of phenolic com-pounds in the saturated range to assure that the sensorresponse is being kinetically controlled by the biomi-metic reaction.
3. Results and discussion
3.1. Copper complex and membrane thickness effects onthe sensor response
In a first step, an experiment carried out in order to
test the response of the unmodified (andmodified GC electrode with a Nafion† membrane(and a Nafion† membrane doped with coppercomplex (indicated clearly the role of thecomplex in sensor preparation. For the redox coupleof copper complex the formal potential was /140 mVversus SCE. The shape of the voltammogram suggeststhat the process is not fully reversible, as the peakseparation is about 105 mV and is almost constant for
Fig. 1. Cyclic voltammograms obtained with (a) a bare GC electrode;
different scan rates (v ). The peak current was propor-
(b) a GC electrode covered with a Nafion† membrane; and (c) a GCelectrode
tional to the v1/2, suggesting that the process is similar to
[Cu(bipy)3]Cl2×/6H2O. Scan rate 10 mV s1.
those controlled by diffusion. Since the copper complexis adsorbed on the electrode surface, the supporting
copper complex, amperometric measurements were
electrolyte should be responsible to keep the electro
carried out at /50 mV in presence of H2O2 and, no
satisfactory response was observed with this electrode,
After that, in order to verify the response of the GC
as can be observed in the curve a of . When the GC
electrode coated with a Nafion† membrane without
electrode was coated with a Nafion† membrane doped
M.D.P.T. Sotomayor et al. / Electrochimica Acta 48 (2003) 855 /865
the sensing membrane, since the complex has animportant role in the film reticulation. Thus, when thecopper concentration increases on the electrode surface,the analyte/product diffusion through the membranebecomes more difficult and the sensor response de-creases. In order to minimize the diffusion effect,membranes prepared using a small amount of complexare more adequate, but should be considered the sensorstability.
For concentrations higher than 3 g dm3 the
obtained membrane was not uniform and the responsewas unstable. Based on this aspect, the membraneprepared with 2 g dm3 copper complex solution waschosen as giving the best sensor performance, assuringthat the complex is homogenously dispersed on the film.
Fig. 2. Amperometric responses for dopamine obtained with GC
The sensor prepared in this way allowed to obtain
electrodes coated with a Nafion† membrane (a) without coppercomplex; (b) with [Cu(bipy)3]Cl2×/6H2O. Applied potential of /50
uniform films, good signals, reproducibility and stabi-
mV vs. SCE, in 0.1 mol dm3 phosphate buffer (pH 7.0).
The influence of the membrane thickness on the
sensor response was also investigated. The results in
with [Cu(bipy)3]Cl2×/6H2O a good reduction current
indicate that there is no significant influence of
density (j ) was observed at /50 mV in the presence of
the membrane thickness on the sensor response, in the
H2O2 (curve b in All these studies suggest that
range between 15 and 46 mm. This behavior suggests
the copper complex is working as a catalyst to the
that the Nafion† film is not influencing in the interac-
reaction between H2O2 and dopamine (or other phenolic
tion of the analyte/biomimetic catalyst (copper com-
compounds) on the sensor surface, and then this is
plex). This aspect is important to assure the same
electrochemically reduced. Based on these characteris-
response in a wide range of thickness (15 /46 mm) giving
tics, the amount of copper complex in the Nafion†
a high reproducibility.
membrane is very important to get larger response.
For thicker membranes the response decreases, prob-
The effect of the copper (II) complex concentration in
ably in this case the Nafion† film generates a barrier
the membrane preparation was investigated.
between the complex and analyte. On the other hand, its
shows the results obtained for the sensor prepared with
adherence on the electrode surface was poor. Thus,
different concentrations of the copper complex in DMF
membranes with 23 mm thickness were chosen, because
solution. It can be observed that, the best response was
allowed to construct sensors with a good sensitivity and
obtained for a copper complex concentration of 1 g
mainly an optimal repeatability in the sensors prepara-
dm3. However, using lower concentrations, lower than
2 g dm3, the sensor was not stable suggesting thatcopper complex aid in the film reticulation. Although,
3.2. Hydrogen peroxide influence
the sensor prepared using a solution of 2 g dm3presents a lower sensitivity, it was much more stable.
shows the results obtained in the experiments
The sensitivity decreases as complex concentration is
carried out in the presence and absence of hydrogen
increased. This might be probably explained, consider-
peroxide. In the absence of H2O2 (curve a) no signal was
ing a compromise between the available amount of
observed, while in the presence of H2O2 (curve b) a good
cupric centers and the diffusion of analyte/product in
response for dopamine was obtained, suggesting that the
Influence of complex concentration used for membrane preparation on
Influence of the membrane thickness on the sensor response in
the sensor sensitivity, in the presence of 150 mmol dm3 H2O2
presence of 150 mmol dm3 H2O2
[Cu(bipy)3]Cl2×/6H2O (g dm3) Sensitivity (nA dm3 mmol1 cm2)
Membrane thickness (mm)
Sensitivity (nA dm3 mmol1 cm2 l)
*Standard deviation for three replicates.
*Standard deviation for three replicates.
M.D.P.T. Sotomayor et al. / Electrochimica Acta 48 (2003) 855 /865
curve a), and the second is the H2O2 reduction catalyzedby the copper complex (c). Thus, initially areduction current is always observed before the phenolsaddition.
In the proposed sensor, the prior addition of hydro-
gen peroxide, before the additions of phenolic com-pounds, can be explained considering the catalyticmechanism of Tyr from Cu4
In the monophenolase cycle (ortho -hydroxylation ofmonophenols), the active site must be oxygenated togenerate the oxy species. In the presence of the substratea series of proton exchanges occur resulting in anoxygen insertion into the phenolic ring and electrondonation from the copper atoms yielding the o -quinone,
Fig. 3. Current density (j ) obtained with the proposed sensor in the
water and the deoxy reduced form, which is oxidized in
absence (a) and presence (b) of H2O2. Applied potential of /50 mV vs.
order to regenerate the Cu4
deoxy form, with the aid
SCE, 0.1 mol dm3 phosphate buffer (pH 7.0).
of an oxidizing agent or by applying an adequate
peroxide is very important in the sensor response. The
potential, in order to bind new H2O2. In the cycle
signal observed after H2O2 addition is due to mainly two
involving catechol oxidation (o -diphenols to o -qui-
contributions; the first one, is the inherent electroche-
nones), the coordination of the catecholic substrate to
mical reduction of H2O2 on the sensor surface ,
the oxy-cupric form results in the breakdown of the
Fig. 4. Mechanism for Tyr in which the oxy species is formed from Cu4
and H2O2, in order to oxidize phenol and catechol compounds. T: tyrosine
and D: DOPA bound forms.
M.D.P.T. Sotomayor et al. / Electrochimica Acta 48 (2003) 855 /865
complex with electron transfer from the catechol to
dence of the current obtained on the measurement
oxygen, giving the corresponding o -quinone and water.
carried out in anaerobic or aerobic conditions
This leaves the copper atoms at the active site in the
The factors that can be affecting the signal are: (1) the
oxidized state (Cu2). In this state, it cannot bind O2 or
possible O2 reduction catalyzed by the [Cu(bipy)3]Cl2×/
H2O2; therefore it is inactive to phenolic substrates. On
6H2O complex; (2) the efficiency of copper-oxy species
the other hand, this form is able to coordinate another
formation; and (3) possibly the spontaneous dopamine
molecule of catecholic substrate and oxidizes it to the o -
oxidation in presence of oxygen at neutral media
quinone by donation of electrons to copper atoms,
but not significant. In order to evaluate the influence of
generating the deoxy-reduced form (Cu2
the measurement's conditions, studies were carried out
In the sensor is expected that [Cu(bipy)3]Cl2×/6H2O
in N2 saturated solutions (absence of O2), air saturated
perform the same role of the Tyr active site, according to
solution (bubbling air) and air equilibrated solution
the mechanism proposed in This mechanism is
(without bubbling any gas).
based on the Tyr enzyme mechanism, which catalyses
shows clearly that in aerobic conditions (air
the oxidation of phenolic compounds through copper-
equilibrated and air saturated solutions) the sensitivities
oxy species generation , and considering that the
are lower than the responses obtained in the anaerobic
copper complexes are dispersed in the Nafion† mem-
condition. This behavior can be explained due to O2
brane in a favorable way to the formation of the Cu4
reduction catalyzed by the [Cu(bipy)3]Cl2×/6H2O, as
sites. In a first step, the hydrogen peroxide
clearly observed in . When the sensor is used in
(or eventually O2) could react directly with two Cu2
aerobic conditions the O2 reduction is catalyzed by the
ions, forming the oxy species, necessary for phenol
complex, competing for the copper active centers with
oxidation to o -quinone species. In this stage the copper
the H2O2. Thus, the copper-oxy species generation is
centers are reduced to Cu. However, an adequate
affected resulting in lower sensitivity observed when
potential should be applied in order to allow the copper
compared with those obtained in anaerobic condition.
oxidation to complete the catalytic cycle, and at the
Although, during the O2 reduction by the complex could
same time the o -quinone might be reduced to catechol
form copper-oxy site a high potential is necessary to
electrochemically. The two electrons left in the copper
reduce O2. The higher sensitivity obtained in anaerobic
oxidation could be used for oxygen reduction in the oxy
condition indicates the importance of hydrogen perox-
species to form H2O and o-quinone. In a following step,
ide in the sensor response mechanism, indicating that
the catechol electrochemically produced, can react with
the intermediate copper-oxy species should be the
another oxy species, forming the o -quinone and pseudo-
responsible for the dopamine oxidation. Thus, the
meta tyrosinase. At this stage, the most important step
sensitivity obtained is a compromise between; (1) the
for phenol quantification, the electrochemical reduction
facility of oxy species formation in O2 presence; and (2)
of the o -quinone could occur on the electrode surface,
the O2 reduction by the complex, diminishing the
and a signal amplification may be obtained due to the
amount of the active cupric centers that could react to
cycle formed, making an analogy with the enzymatic
form the oxy species.
systems for catecholamines detection The same
In the present work, the measurements were carried
mechanism is reported for many amperometric biosen-
out in air equilibrated solution, which is more practical
sors based on the Tyr enzyme Nevertheless, the
for applications, because the sensitivity in this condition
cathodic current observed will be proportional to the
is enough to detect dopamine in the desired samples.
phenol concentration. In order to complete the catalyticcycle, another catechol species could reacts with the
3.4. Influence of the applied potential
pseudo-meta form, generating o -quinone and the Cu.
Copper is electrochemically oxidized to complete the
In is clearly seen that the sensor presents best
catalytic cycle, and these two electrons are used to
response at /50 mV versus SCE. This result is a
reduce the o -quinone formed. This last cycle always
compromise between the optimum potential for electro-
allow the conversion of pseudo-meta species to reduced
chemical reduction of quinone species to dopamine, and
copper in order to complete the catalytic cycle.
the peroxide consumption at more negative potentials,
Considering this mechanism and the obtained results
through its electrochemical reduction Thus, in
in the evaluation of the influence of peroxide concentra-
more negative potentials the amount of H2O2 available
tion on the sensor response the concentration of
to form the reactive oxy species diminishes, due to the
150 mmol dm3, was used in all further experiments.
inherent electrochemical reduction of H2O2; conse-quently the sensitivity is lower. It is also important
3.3. Measurements conditions
that the copper be in oxidized form to present catalyticproperty, thus the potential cannot be much negative to
In the development of this amperometric sensor for
avoid the copper reduction. For higher potentials the
phenolic compounds is observed that there is a depen-
possibility of o -quinone species reduction is minimized.
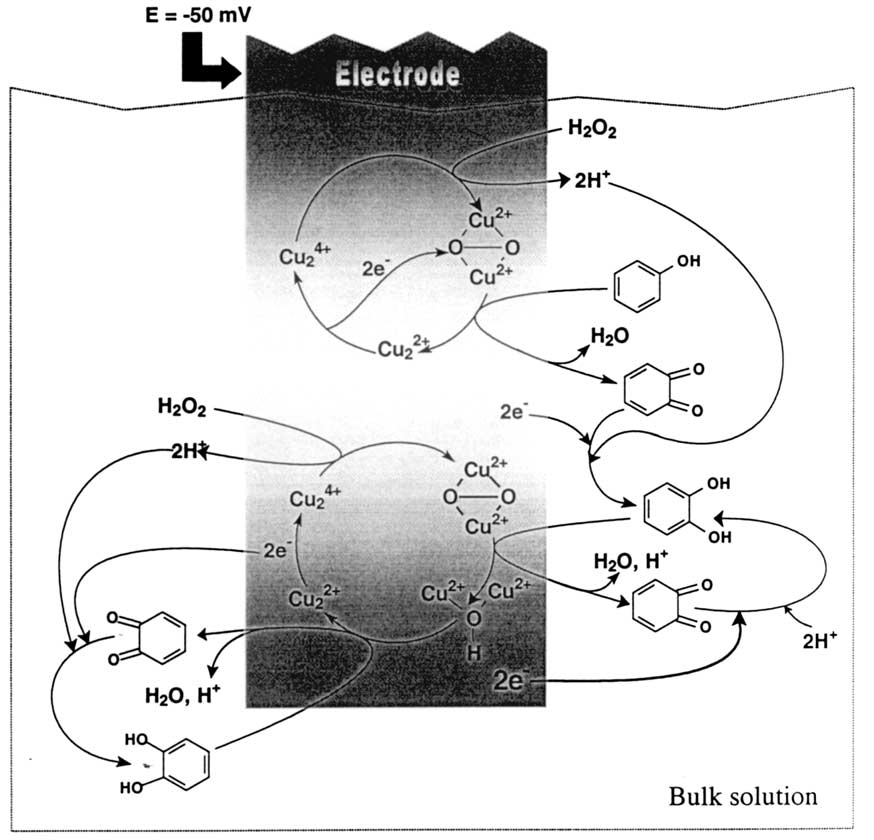
M.D.P.T. Sotomayor et al. / Electrochimica Acta 48 (2003) 855 /865
Fig. 5. Proposed mechanism for dopamine and other phenolic compound oxidation for the sensor modified with [Cu(bipy)3]Cl2×/6H2O.
Fig. 7. Profile of the sensor response, in aerobic and anaerobic media,
Fig. 6. Dependence of H2O2 concentration on the current density (Dj)
for dopamine. Applied potential of /50 mV vs. SCE, in 0.25 mol
obtained with the proposed sensor. Applied potential of /50 mV vs.
dm3 Pipes buffer (pH 7.0) containing 150 mmol dm3 H2O2.
SCE, in 0.1 mol dm3 phosphate buffer (pH 7.0) containing 100 mmoldm3 of dopamine.
influence of the Pipes buffer concentration on the sensorresponse showed that the best result was obtained in a
3.5. Influence of the pH, buffer and buffer concentration
concentration of 0.25 mol dm3
The investigation to evaluate the pH effect on the
3.6. Sensor characteristics
sensor response showed an optimum pH at 7.0 (in 0.1 mol dm3 phosphate buffer solution. Experi-
In the optimized conditions the proposed sensor
ments carried out in three different buffer solutions
showed a linear response range from 9.5 up to 230
(in a concentration of 0.10 mol dm3, indicated
mmol dm3 (which can be expressed according
that Pipes buffer gives the best response. Finally, the
to the following equation:
M.D.P.T. Sotomayor et al. / Electrochimica Acta 48 (2003) 855 /865
37(92)1:43(90:01) [DA]=mmol dm3
with a correlation coefficient of 0.999, for n /22. Theprofile of the full curve showed that the sensor responseis linear at low concentrations of dopamine and becomesindependent at higher dopamine concentration (data notshown). This result provides a mechanistic indicator thatthe dopamine oxidation indeed follows Michaelis /Menten kinetics such as occurs in enzymaticbiosensors.
The detection limit of 4.8 mmol dm3 was calculated
according to Serra et al. The response time,
Fig. 8. Cyclic voltammograms obtained with the proposed sensor in
considering the time to reach 100% of the signal, was
0.25 mol dm3 Pipes buffer (pH 7.0) in (a) N2 saturated solution and
approximately 1 s. The response time showed by this
(b) after the saturation with air (dot line). Scan rate 10 mV s1.
Table 3Influence of the buffer on the current density (Dj ) obtained for theproposed sensor for 110 mmol dm3 dopamine, in presence of 150mmol dm3 H2O2
Buffer (0.1 mol dm3)
*Standard deviation for three replicates.
Table 4Influence of the pipes concentration on current density (Dj ) for 100mmol dm3 dopamine, in presence of 150 mmol dm3 H2O2
[Pipes] (mol dm3)
Fig. 9. Influence of the applied potential on the sensor response.
Measurements carried out in 0.1 mol dm3 phosphate buffer (pH 7.0)
containing 100 mmol dm3 of dopamine and 150 mmol dm3 of H2O2.
*Standard deviation for three replicates.
Fig. 10. Response profile for the sensor in phosphate buffer solutionswith different solution pH. Applied potential of /50 mV vs. SCE, in
Fig. 11. A typical profile of the sensor response in the optimized
0.1 mol dm3 phosphate buffer containing 100 mmol dm3 of
conditions. Applied potential /50 mV vs. SCE, in 0.25 mol dm3
dopamine and 150 mmol dm3 of H2O2.
Pipes buffer solution (pH 7.0), containing 150 mmol dm3 of H2O2.
M.D.P.T. Sotomayor et al. / Electrochimica Acta 48 (2003) 855 /865
Table 5Analytical and kinetic parameters of the proposed sensor for dopamine and analogous compounds, in the optimised conditions
sensor was better than the other sensors presented in the
as R.S.D. was lower than 5%. This result indicates a
literature for phenolic compounds .
good repeatability in the sensor construction.
The repeatability in the measurements was evaluated
This sensor can be kept at room temperature and
through thirteen successive experiments carried out with
used, without loosing the sensitivity, after several
30 mmol dm3 dopamine solutions. The repeatability
months. The good stability is probably due to the
was evaluated as the relative standard deviation (R.S.D)
elimination of enzyme denaturation problems caused
giving a value of 4.8%.
by time and temperature.
Repeatability in the sensors construction was evalu-
Under operational conditions the proposed sensor
ated preparing four sensors and determined the sensi-
presented good stability during more than 150 determi-
tivity obtained for each one. The repeatability expressed
nations, as shown in
Fig. 12. Relative response (%) as a function of the number ofdeterminations. The parameter was calculated considered the sensorresponse in the first determination as 100%. Applied potential of /50
Fig. 13. Eadie /Hofstee plot, obtained for the dopamine using the
mV vs. SCE, in 0.1 mol l1 phosphate buffer (pH 7.0) containing 100
proposed sensor, in which the [Cu(bipy)3]Cl2×/6H2O is a biomimetic
mmol l1 of dopamine and 150 mmol l1 of H2O2.
catalyst of the Tyr enzyme.
M.D.P.T. Sotomayor et al. / Electrochimica Acta 48 (2003) 855 /865
3.7. Effect of the phenolic substrates
Table 7Determination of dopamine in pharmaceutical preparations
In this work a detailed study was carried out, in order
Dopamine containing in 10.0 ml of sample (mg)
to investigate the sensor response for other phenoliccompounds besides dopamine, including: aromatic
amines, nitro-phenols, catecholic compounds and neu-
rotransmitters. Among the eighteen compounds evalu-
ated, the proposed sensor presented response only for
Standard deviation for three replicates.
b Standard deviation for five replicates.
five of them. These compounds are; dopamine (DA),catechol (CAT), norepinephrine (NE), 4-aminophenol
the high responses observed for 4-APh and p -PhA, and
(4-APh) and p -phenylenediamine (p -PhA). The last two
lower response obtained for DA, CAT and NE.
compounds presented responses 8.4 and 5.4 times,respectively, higher than that observed for dopamine.
In is shown the analytical and kinetics
parameters obtained for the compounds with structure
3.8. Effect of interfering compounds
very similar to dopamine. It can be observed that the
The sensor response was tested in presence of 130
dopamine presented best sensitivity and linear response
mmol dm3 dopamine and compounds such as uric acid,
range, but slightly smaller affinity than catechol; this
ascorbic acid and acetaminophen, and other compounds
last parameter was evaluated through the value of the
commonly contained in the pharmaceutical formula-
apparent Michaelis /Menten constant. Although, this
tions, such as sodium chloride and sodium bisulfite, in
constant is commonly defined for enzymatic systems,
different levels of molar ratios. The results obtained with
this kinetic parameter is being used evidently by
the proposed sensor () showed no significant
analogy. The used of the Eadie /Hofstee form is quite
interference in presence of the investigated compounds.
efficient in the kinetics analysis because, it could provide
Ascorbic acid gave some small interference at a molar
an instrument to discriminate kinetics from diffusion
ratio of 1:1, presumably due to reaction with hydrogen
process. The linear plot in (correlation coeffi-
peroxide. However, as the sensor response is indepen-
cient 0.992 for n /17) shows that apparently it is
dent for concentrations of H2O2 higher than 150 mmol
kinetically controlled, and the biomimetic reaction is
dm3, it could be overcome using more H2O2.
the rate controlling process. Thus, this result is otherevidence that the mechanism proposed in iscoherent.
The sensitivity and selectivity order observed for the
studied compounds can be explained based on their
The applicability of the amperometric sensor in the
redox potential, as it stands with respect to the use of
dopamine determination was evaluated, on pharmaceu-
metal complex. It is know that hydroquinone and other
tical samples. In the analyzed sample containing in its
analogous compounds such as 4-APh and p -PhA, are
formulation preservatives and salts, such as the anti-
easily and rapidly oxidized Their oxidation poten-
oxidant sodium bisulfite no significant interference
tials are lower than the ortho -analogous compounds,
was observed. The results (were compared
such as catechol. The facility for oxidation diminished,
favorably with those obtained with the USP official
respectively, for resorcinol, 2-nitrophenol, 3-nitrophe-
method showing that the result is statistically the
nol, 4-nitrophenol and phenol This could explain
same with 95% of confidence.
Table 6Recovery (%) obtained for 130 mmol dm3 dopamine in presence of different interfering compounds in various levels of molar ratios
Molar ratio interfering: dopamine
*Standard deviation for three replicates; n.e., non evaluated.
M.D.P.T. Sotomayor et al. / Electrochimica Acta 48 (2003) 855 /865
[14] R. Breslow, Acc. Chem. Res. 13 (1980) 170.
[15] R. Breslow, L.E. Overman, J. Am. Chem. Soc. 92 (1970) 1075.
[16] J. Costamagna, G. Ferraudi, B. Matsuhiro, M. Campos-Vallette,
This work clearly shows that by using a simple
J. Canales, M. Villagra´n, J. Vargas, M.J. Aguirre, Coord. Chem.
compound with biomimetic chemistry in the construc-
Rev. 196 (2000) 125.
tion of amperometric sensor is possible efficiently to
[17] M.D.P.T. Sotomayor, L.T. Kubota, Quim. Nova 25 (2002) 123.
detect dopamine and other analogous compounds. This
[18] M.D.P.T. Sotomayor, A.A. Tanaka, L.T. Kubota, Anal. Chim.
sensor showed a wide linear response range, good
Acta 455 (2002) 215.
[19] S. Berchmans, H. Gomathi, G.P. Rao, Sens. Actuat. B 50 (1998)
sensitivity, excellent stability and good repeatability.
The higher sensitivities obtained for diphenols com-
[20] Y. Hasebe, T. Akiyama, T. Yagisawa, S. Uchiyama, Talanta 47
pound and no signal for monophenols, is a good
(1998) 1139.
indication that the [Cu(bipy)
[21] A.A. Karyakin, E.E. Karyakina, Sens. Actuat. B 57 (1999) 268.
3]Cl2 ×/H2O complex immo-
bilized in the Nafion† membrane is mimicking the Tyr
[22] R. Gupta, R. Mukherjee, Tetrahedron Lett. 41 (2000) 7763.
[23] M. Maumy, P. Capdevielle, J. Mol. Catal. A: Chem. 113 (1996)
active site.
[24] J. Gao, S.H. Zhong, J. Mol. Catal. A: Chem. 164 (2000) 1.
[25] J. Gao, S.H. Zhong, J. Mol. Catal. A: Chem. 168 (2001) 1.
[26] R. Raja, P. Ratnasamy, Appl. Catal. A 143 (1996) 145.
[27] R. Raja, P. Ratnasamy, J. Mol. Catal. A: Chem. 100 (1995) 93.
[28] E.I. Solomon, Chem. Rev. 96 (1996) 2563.
The authors acknowledge financial support from
[29] P.A. Riley, J. Theor. Biol. 203 (2000) 1.
Fundac¸a˜o de Amparo a Pesquisa do estado de Sa˜o
[30] D.E. Wilcox, A.G. Porras, Y.T. Hwang, K. Lerch, M.E. Winkler,
Paulo (FAPESP). M.D.P.T.S. is indebted to FAPESP
E.I. Solomon, J. Am. Chem. Soc. 107 (1985) 4015.
for a fellowship.
[31] R.J. Meyer, Gmelins Handbuch der Anorganishen Chemie,
Springer-Verlag, Berlin, 1958, p. 311.
[32] R.A. Palmer, T.S. Piper, Inorg. Chem. 5 (1966) 564.
[33] P. Calvo-Marzal, S.S. Rosatto, P.A. Granjeiro, H. Aoyama, L.T.
Kubota, Anal. Chim. Acta 441 (2001) 207.
[34] J. Langmaier, A. Troja´nek, J. Weber, Z. Samec, J. Electroanal.
[1] S. Zhang, H. Zhao, R. John, Anal. Chim. Acta 441 (2001) 95.
Chem. 469 (1999) 11.
[2] S. Cosnier, J.J. Fombon, P. Labbe´, D. Limosin, Sens. Actuat. B
[35] R.A. Kamin, G.S. Wilson, Anal. Chem. 52 (1980) 1198.
59 (1999) 134.
[36] M. Fontecave, J.-L. Pierre, Coord. Chem. Rev. 170 (1998) 125.
[3] C. Capannesi, I. Palchetti, M. Mascini, A. Parenti, Food Chem.
[37] F. Lisdat, U. Wollenberger, A. Makower, H. Ho¨rtnagl, D.
71 (2000) 553.
Pfeiffer, F.W. Scheller, Biosens. Bioelectron. 12 (1997) 1199.
[4] C. Nistor, J. Emne´us, L. Gorton, A. Ciucu, Anal. Chim. Acta 387
[38] L. Coche-Gue´rente, V. Desprez, J.-P. Diard, P. Labbe´, J.
Electroanal. Chem. 470 (1999) 53.
[5] P. Dantoni, S.H.P. Serrano, A.M.O. Brett, I.G.R. Gutz, Anal.
[39] E. Herlinger, R.F. Jameson, W. Linert, J. Chem. Soc., Perkin
Chim. Acta 366 (1998) 137.
Trans. 2 (1995) 259.
[6] M. Hedenmo, A. Narva´ez, E. Domı´nguez, I. Katakis, J. Electro-
[40] J.M. Zen, A.S. Kumar, M.R. Chang, Electrochim. Acta 45 (2000)
anal. Chem. 425 (1997) 1.
[7] M. Pravda, C. Petit, Y. Michotte, J.-M. Kauffmann, K. Vytras, J.
[41] B. Serra, B. Benito, L. Agu¨ı´, A.J. Reviejo, J.M. Pingarro´n,
Chromatogr. A 727 (1996) 47.
Electroanalysis 13 (2001) 693.
[8] M. Lutz, E. Burestedt, J. Emne´us, H. Lide´n, S. Gobhadi, L.
[42] L. Rover, Jr., J.C.B. Fernandes, G. Oliveira-Neto, L.T. Kubota,
Gorton, G. Marko-Varga, Anal. Chim. Acta 305 (1995) 8.
J. Electroanal. Chem. 481 (2000) 34.
[9] A.W.O. Lima, E.K. Vidsiunas, V.B. Nascimento, L. Angnes,
[43] B. Wang, S. Dong, J. Electroanal. Chem. 487 (2000) 45.
Analyst 123 (1998) 2377.
[44] H. Musso, in: W.I. Taylor, A.R. Battersby (Eds.), Oxidative
[10] A.W.O. Lima, V.B. Nascimento, J.J. Pedrotti, L. Angnes, Anal.
Coupling of Phenols, Marcel Decker, New York, 1967, p. 58.
Chim. Acta 354 (1997) 325.
[45] M.L. Mihailovic, Z. Cekovic, in: S. Patai (Ed.), The Chemistry of
[11] F. Ortega, E. Domı´nguez, G.J. Peterson, L. Gorton, J. Biotech-
Hydroxyl Group (Part 1), Wiley-Interscience, New York, 1971, p.
nol. 31 (1993) 289.
[12] F. Ortega, E. Domı´nguez, E. Burestedt, J. Emne´us, L. Gorton, G.
[46] P. Nagaraja, K.C.S. Murthy, K.S. Rangappa, N.M.M. Gowda,
Marko-Varga, J. Chromatogr. A 675 (1994) 65.
Talanta 46 (1998) 39.
[13] F. Ortega, J.L. Cuevas, E. Domı´nguez, J. Pharm. Biomed. Anal.
[47] United States Pharmacopeia National Formulary XXI, US
10 (1992) 789.
Pharmacopeial Convention, Rockville, MD, 1985, p. 353.
Source: http://www.unifacs.br/upload/recat_docs/Tris_2,2-bipyridil_%20copper_II_chloride%20complex%20a%20biomimetic.pdf
ÁLVarez-taFur, M. la Decisión 391 de 1996 de la Comunidad Andina: su lectura antes y después del tlC de Colombia con Estados Unidos en materia de acceso y protección de los recursos genéticos y biológicos* Andean Community Decision 391, of 1996: reading before and after the FTA between Colombia and the United States on access
William J. Faulkner, M.D. Dr. Faulkner is a consultant for : Al ergan Bausch & Lomb Physicians Recommended Nutriceuticals Allergy: An Altered Immune Response From the Greek words "allos" meaning different or And "ergos" meaning work or action…an "altered Normal response to exposure to foreign substance is production of IgM, IgA, IgG and elimination of foreign