Effects of substrate stiffness on cell morphology, cytoskeletal structure, and adhesion
Cell Motility and the Cytoskeleton 60:24 –34 (2005)
Effects of Substrate Stiffness on Cell
Morphology, Cytoskeletal Structure,
Tony Yeung,1 Penelope C. Georges,1 Lisa A. Flanagan,2 Beatrice Marg,2
Miguelina Ortiz,1 Makoto Funaki,1 Nastaran Zahir,1 Wenyu Ming,1
Valerie Weaver,1 and Paul A. Janmey1,2*
1
Institute for Medicine and Engineering, University of Pennsylvania, Philadelphia
2
Hematology Division, Brigham and Women's Hospital, Boston, Massachusetts
The morphology and cytoskeletal structure of fibroblasts, endothelial cells, andneutrophils are documented for cells cultured on surfaces with stiffness rangingfrom 2 to 55,000 Pa that have been laminated with fibronectin or collagen asadhesive ligand. When grown in sparse culture with no cell-cell contacts, fibro-blasts and endothelial cells show an abrupt change in spread area that occurs at astiffness range around 3,000 Pa. No actin stress fibers are seen in fibroblasts onsoft surfaces, and the appearance of stress fibers is abrupt and complete at astiffness range coincident with that at which they spread. Upregulation of ␣5integrin also occurs in the same stiffness range, but exogenous expression of ␣5integrin is not sufficient to cause cell spreading on soft surfaces. Neutrophils, incontrast, show no dependence of either resting shape or ability to spread afteractivation when cultured on surfaces as soft as 2 Pa compared to glass. The shapeand cytoskeletal differences evident in single cells on soft compared to hardsubstrates are eliminated when fibroblasts or endothelial cells make cell-cellcontact. These results support the hypothesis that mechanical factors impactdifferent cell types in fundamentally different ways, and can trigger specificchanges similar to those stimulated by soluble ligands. Cell Motil. Cytoskeleton60:24 –34, 2005.
2004 Wiley-Liss, Inc.
Key words: substrate stiffness; cell morphology; fibroblasts; mechanosensing; cell-matrix interaction;
actin cytoskeleton; integrin expression
transcriptional changes mediating cells' responses tostiffness are beginning to be characterized.
Most cells in multicellular organisms are at-
Previous studies using fibroblasts have shown that
tached to much softer materials than the glass and
cells generate more traction force and develop a broader
plastic surfaces on which nearly all studies are done in
and flatter morphology on stiff substrates than they do on
vitro. The most common attachment site for a mam-malian cell is another similar cell or the extracellularmatrix, and these materials have elastic moduli on the
order of 10 to 10,000 Pa [Bao and Suresh, 2003;
Correspondence to: Paul Janmey, Institute for Medicine and Engineering
(IME), University of Pennsylvania, 1010 Vagelos Laboratories, 3340
Wakatsuki et al., 2000]. Forces generated by cytoskel-
Smith Walk, Philadelphia, PA 19104.
etal motors applied to membrane attachment sites can
deform materials with this range of stiffness but can-not move an attachment site on a rigid surface. Con-
Received 22 April 2004; accepted 27 August 2004
sequently, cell morphology and functions can depend
Published online in Wiley InterScience (www.interscience.wiley.
strongly on substrate stiffness under conditions where
chemical signals are constant. The acute signal and
DOI: 10.1002/cm.20041
2004 Wiley-Liss, Inc.
Cell Morphology and Substrate Stiffness
soft but equally adhesive surfaces and that cells will
Preparation of Polyacrylamide Gel
preferentially migrate from a soft to a hard surface [Lo et
A 30% w/v acrylamide stock solution is prepared
al., 2000]. Cell growth and survival also depend on
by mixing 15 g of acrylamide powder (FisherBiotech
substrate stiffness, as gelatin-coated materials softer than
CAS no. 79061) with 35 mL of deionized H O. A
100 Pa do not support survival of non-transformed cells
1% w/v bis-acrylamide stock solution is prepared by
but do support growth of transformed cell types [Wang et
mixing 500 mg bis-acrylamide powder (FisherBiotech
al., 2000a]. Large changes in the extent of protein ty-
CAS no. 110269) with 49.5 mL H O. Polyacrylamide gel
rosine phosphorylation and cytoskeletal structure corre-
solutions are prepared with acrylamide at final concen-
late with stiffness, and are presumably part of the stiff-
trations of 3, 5, 7.5, or 12% w/v and bis-acrylamide from
ness-sensing apparatus [Pelham and Wang, 1997].
0.05 to 0.6% w/v. To polymerize the solution, 1.5 L
Responses to mechanical stimuli may be cell-type spe-
TEMED (FisherBiotech CAS no. 110189) and 5 L of
cific. Motor neurons derived from embryonic mouse
10% ammonium persulfate are added with the appropri-
spinal cord extend neurites with extensive branches on
ate amount of H O to yield a final volume of 1,000 L.
soft but not hard surfaces [Flanagan et al., 2002]. In
A fixed volume of 45 L of the polyacrylamide solution
contrast, smooth muscle cells, like fibroblasts, extend
is immediately pipetted onto the center of the 25-mm-
processes more avidly on hard surfaces and are rounded
diameter glass cover slip. The 18-mm-diameter cover
on soft materials [Engler et al., 2004].
slip is then carefully placed on top of the polyacrylamide
In this study, we extend the range of cell types
solution. The polymerization is completed in about
examined on materials with well-controlled stiffness by
10 min and the top coverslip is slowly peeled off. The
employing protein-laminated polyacrylamide gels to
bottom cover slip with the attached polyacrylamide gel is
study GFP-actin and GFP-␣5 integrin-expressing NIH
immersed in a multi-well plate (6 Well Cell Culture
3T3 fibroblasts, bovine aorta endothelial cells, and hu-
Cluser, Costar 3516, Corning Inc., Corning, NY) with
man neutrophils. The results show that the morphologies
3 mL PBS. Polyacrylamide gels with a wide range of
of different cell types differ both quantitatively and qual-
shear storage moduli, G⬘, can be prepared using the
itatively with substrate stiffness, that the stiffness re-
concentration scheme described above. The most flexible
sponse depends on the nature of the adhesion ligand
gel with 3% w/v acrylamide and 0.05% w/v bis-acryl-
bound to the surface, and that changes in stiffness can
amide has a shear elastic modulus (G⬘) of 2 Pa while the
initiate specific transcription events leading to upregula-
stiffest gel with 12% w/v acrylamide and 0.60% w/v
tion of adhesion receptors.
bis-acrylamide yields a G⬘ of 55,000 Pa.
MATERIALS AND METHODS
Crosslinking of Adhesion Proteins
Preparation of Glass Cover Slips
A heterobifunctional crosslinker, sulfo-SANPAH
The substrate is prepared by allowing polyacryl-
amide solutions to polymerize between two chemically
anoate, Pierce no. 22589), is used to crosslink extracel-
modified glass cover slips. Briefly, 200 L of a 0.1 N
lular matrix molecules onto the surface of the gel. A
NaOH solution is pipetted to cover the surface of a
small amount (about 1 mg/ml) of sulfo-SANPAH is
25-mm-diameter glass cover slip (Fisherbrand, catalog
dissolved in H O, and 200 L of this solution is pipetted
no. 12-545-102; Fisher Scientific, Pittsburgh, PA) for
onto the gel surface. The polyacrylamide gel is then
5 min. The NaOH solution is then aspirated, and 200 L
placed 6 inches under an ultraviolet lamp and irradiated
of 3-APTMS (3-Aminopropyltrimethoxysilane, Sigma
for 10 min. It is then washed three times each with 3 mL
no. 28-1778, Sigma, St. Louis, MO) is applied for 3 min.
of 200 mM HEPES at pH 8.6. After the last HEPES
The glass cover slip is thoroughly rinsed with de-ionized
solution is aspirated, 200 L of a 0.14 mg/ml fish fi-
water to wash away any remaining 3-APTMS solution.
bronectin solution (Sea Run Holdings, South Freeport,
Then, 200 L of 0.5%v glutaraldehyde (Sigma no.
ME) [Wang et al., 2000b] or 0.14 mg/ml type I collagen
G7651) in H O is added onto the cover slip for 20 min.
is pipetted on top of the polyacrylamide gel. The multi-
The glass cover slip is subsequently rinsed with water.
well plate housing the gels is then incubated at 5°C for at
An 18-mm-diameter glass cover slip is placed on top of
a piece of parafilm inside a tissue culture dish. A small
amount of a 10% by volume Surfasil solution (Pierce no.
42800, Pierce, Rockford, IL) in chloroform is pipetted
The viscoelastic properties of polyacrylamide gels
onto the parafilm near the cover slip. The tissue culture
were quantified by measuring the dynamic shear moduli
dish with a half-closed lid is placed inside a vacuum
using an RFS II fluids spectrometer (Rheometrics Inc.,
desiccator for 10 min.
Piscataway, NJ). A 500-L sample was polymerized
Yeung et al.
between two 25-mm stainless steel parallel plates with a
chamber using a Bio-Rad Econo-Column pump (Bio-
corresponding sample thickness of approximately 1 mm.
Rad, Richmond, CA). Microscopy began within 3 min
The shear storage modulus G⬘, corresponding to the
after flow was stopped. 3T3 fibroblasts were allowed to
elastic resistance of the gels, was determined from the
grow on either a 180- or 55,000-Pa gel. Cells on the
shear stress in phase with an oscillatory (1 rad/s) shear
55,000-Pa gel were incubated with CO
strain of 2% maximal amplitude, by standard techniques.
medium (GibcoBRL Cat No. 18045-088 Lot No.
Similar measurements were conducted using cone and
1099754) with 10% bovine calf serum to maintain phys-
plate geometries where the thickness of the sample was
iological pH in the absence of a rich CO environment.
between 50 –200 m, similar to that of gels on which
Cells on the 180-Pa gel were incubated with 50% CO2
cells are grown, and nearly identical G⬘ were recorded
independent medium, 40% DMEM, and 10% bovine calf
(data not shown).
serum. The rate of cell spreading was analyzed by takingimages of sparsely distributed cells at 30-sec intervals.
Cell Culture
GFP-actin expressing NIH-3T3 mouse fibroblasts
Quantification of Integrin Expression
prepared as previously described [Cunningham et al.,
NIH 3T3 GFP-actin expressing fibroblasts grown
2001] were incubated in Dulbecco's Modified Eagle Me-
on polyacrylamide gels with varying stiffnesses for 24 h
dium (Cellgro no. 10-013CV) with high glucose, L-
were used for the quantification of ␣5 integrin expression
glutamine, no sodium pyruvate, phenol red, and 10%
by Western blotting. Polyacrylamide gels on top of the
bovine calf serum (Hyclone Cat No. SH30072.03 Lot
25-mm glass cover slips were gently scraped off in one
No. AJH10711 Bottle No. 1007, Hyclone, Logan, UT) at
piece by a razor blade. The gels were immersed in a
a 5% CO environment.
Laemmli lysis buffer solution (50 mM Tris-HCL, pH 6.8,
Bovine aortic endothelial cells were kindly pro-
5 mM EDTA, 2% SDS containing 1 mM sodium fluo-
vided by Peter Davies, and incubated in DMEM with
ride, 1 mM sodium orthovanadate, and a cocktail of
high glucose, L-glutamine, no sodium pyruvate, phenol
protease inhibitors) in an Eppendorf tube for 15 min. The
red, and 10% bovine calf serum at a 5% CO environ-
cell lysate solution with the addition of non-reducing
buffer was then run on an 8% SDS-PAGE gel. The
Human blood neutrophils were isolated from whole
electrophoresis gel was then transferred and blocked
blood from healthy volunteers. Collected blood samples
using standard protocols. The primary antibody used was
were immediately transferred to sodium-heparin-contain-
␣5 integrin, rabbit sera (Chemicon International, Te-
ing polypropylene tubes. Neutrophils were isolated by
mecula, CA), and the secondary used was a horseradish
centrifuging 3 mL whole blood through 3 mL Mono-Poly
peroxidase-linked anti-rabbit IgG polyclonal antibody
resolving media (ICN Biomedical, Irvine, CA) to resolve
(Amersham Pharmacia Biotech, Arlington Heights, IL).
a distinct neutrophil layer. Neutrophils were washed
Protein was detected with an ECL-Plus system (Amer-
two times with Dulbecco's phosphate-buffered saline
sham Pharmacia Biotech). In order to normalize the
(DPBS) (Gibco, Gaithersburg, MD), and contaminating
Western blot result by the number of cells per sample,
erythrocytes lysed by 40-sec incubation in sterile dis-
microscopy was performed to estimate the number of
tilled water. Final neutrophil pellets were resuspended in
cells grown on each gel substrate before the Western blot
RPMI 1640 medium (Gibco, Gaithersburg, MD) and
procedure. The intensity value of each band after sub-
kept on ice prior to plating on substrates.
traction of the background was then normalized by thenumber of cells estimated in each sample.
Phase contrast and fluorescence microscopy were
conducted using a Leica DM IRBE microscope and a
The ␣5 integrin EGFP fusion (generous gift of Rick
Hamamatsu C-4742 digital camera. The multi-well plate
Horowitz, University of Virginia) [Laukaitis et al., 2001]
was briefly removed from the tissue culture incubator for
was excised as a XhoI-NotI fragment from pEGFP-N3
microscopy work. Area and circumference measure-
(Clontech, Palo Alto, CA) and subcloned into the retro-
ments were obtained by tracing cell boundaries manually
viral vector Hermes HRS puro GUS (generous gift of
using NIH Image software.
Helen Blau, Stanford University, CA) [Rossi et al., 1998]replacing the GUS cDNA between SalI and NotI sites
using standard techniques and bringing the fusion under
control of a tetracycline (tet) regulated promoter. To
FCS2 Chamber) was used for time-lapse movies to main-
produce retrovirus, 3 ⫻ 106 HEK 293 cells were seeded
tain temperature at 37°C. Two milliliters of a 10,000 cell/
in gelatin-coated 60-mm dishes, and 24 h later the
ml solution were injected into the temperature-controlled
Hermes HRS puro ␣5 integrin EGFP construct was co-
Cell Morphology and Substrate Stiffness
transfected with pVSVG and pCgp (generous gift ofAlan Kingsman, Oxford University, UK), which expressthe VSVG protein and gag-pol genes, respectively, usingcalcium phosphate. Transfected cells were incubated 8 hat 37°C, media was replaced and returned to 37°C for afurther 16 h, after which they were transferred to a 32°Chumidified incubator. After 24 h at 32°C media wascollected, made 8 g/ml in polybrene (Sigma), and cen-trifuged at 3,000
g for 5 min to pellet cells and debris.
NIH-3T3 cells in a 6-cm dish were incubated with 1.5 mlof retrovirus containing supernatant at 32°C for 8 h, afterwhich the supernatant was replaced with 3 ml of regularmedia and returned to a 37°C humidified incubator for36 – 48 h. Retrovirally transduced cells were selected inmedia supplemented with 1 g/ml puromycin until a
Mechanical properties of polyacrylamide substrates. The
resistant population grew out. Polyclonality was judged
shear modulus of polyacrylamide gels with a range of acrylamide
visually by the number of puromycin-resistant colonies
(indicated as percents near data lines) to bis-acrylamide (indicated as
and speed of outgrowth, and only pooled populations
crosslinker) proportions was measured. The shear modulus (G⬘), ex-
deemed sufficiently polyclonal were used in experiments.
pressed in Pascal, increases at constant polymer mass with increasing
Cells from the pooled population were subsequently in-
crosslinker. Increasing the concentration of acrylamide from 3 to 12%also creates a large stiffness range from 10 to 50,000 Pa. The
solid line
fected as above with a high titer MFG-based retrovirus
denotes the theoretical stiffness of a rubberlike network if every
that expresses the tet repressor fused to the HPV16
crosslink was elastically effective.
activation domain (a derivative of the tet off transactiva-tor construct provided by Helen Blau [Rossi et al., 1998],generated in the laboratory of V. Weaver, unpublished
is varied. Gels with a concentration above 7.5 % in partic-
data, which has wild type DNA binding activity), pre-
ular show a remarkably linear dependence of elastic mod-
pared by transfection in 293GPG cells (generous gift of
ulus on crosslinker concentration spanning nearly two or-
Richard Mulligan, Whitehead Institute, MIT, Cambridge,
ders of magnitude in stiffness. For crosslinked rubberlike
MA) as described in Ory et al. [1996]. Transduced cells
networks like these acrylamide gels, the elasticity is theo-
were maintained in 1 g/ml tetracycline to keep ␣5
retically predicted to be related to the concentration of
integrin EGFP in the uninduced state. Expression of
crosslinks by the relation
␣5 integrin EGFP was induced in cells by culturing in the
absence of tetracycline in medium containing Tet SystemApproved FBS (Clontech) for 48 h prior to experiments.
where n is the number of crosslinks per volume V, and Ris the gas constant [Flory, 1953]. This theoretical limit,
EGFP Expression Analysis
shown by the solid line in Figure 1, is very close to the
Cells were directly fixed using 2% paraformalde-
experimental values measured at high total [polyacryl-
hyde and visualized using a scanning confocal laser
amide] where each bisacrylamide subunit is most likely
(model 2000-MP; Bio-Rad Laboratories) attached to a
to make an elastically effective crosslink.
fluorescence microscope (Nikon Eclipse TE-300). Con-
Figure 2 shows the large dependence of fibroblast
focal images were recorded at 120⫻.
morphology on FN-coated gel stiffness. Previous studieshave shown that the density of adhesion protein bound tothe gel surface is independent of gel stiffness [Flanagan
et al., 2002], and imaging of the gel surface with fluo-
The large range of physiologically relevant stiffness
rescently labeled FN showed a constant amount of pro-
that can be produced using polyacrylamide gels is shown in
tein bound to both the softest and stiffest gels and a
Figure 1. The softest gels that can reproducibly be formed
uniformity of coverage that was at least as good as that
and handled for cell studies are made with 3% acrylamide.
on plastic (data not shown). The shapes of NIH3T3
At low crosslinker concentrations, gels can be produced
fibroblasts after one day in culture range from round,
with elastic moduli below 10 Pa, the consistency of mucus.
nearly spherical cells with a few irregular protrusions
The upper limit to 3% gels appears to be near 600 Pa.
seen on gels of 180 Pa stiffness to large spread cells on
Higher moduli require greater concentrations of acrylamide,
gels stiffer than 16,000 Pa, which were indistinguishable
and as seen in Figure 1, 5.5 and 7.5% gels allow large
from those grown on glass or the plastic surfaces sur-
variations of elastic moduli as the crosslinker concentration
rounding the gel in the culture dish. As visualized by
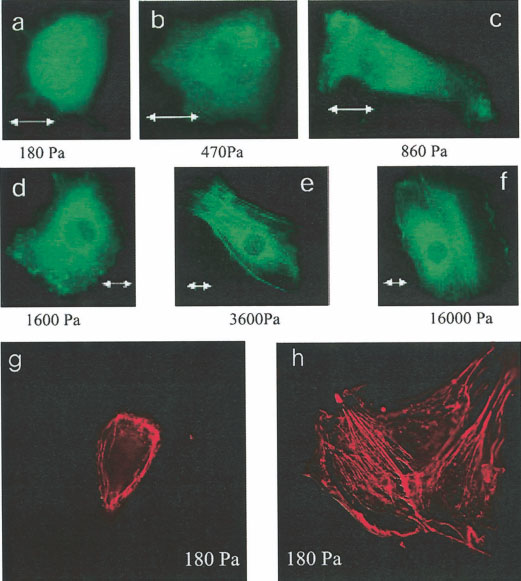
Yeung et al.
Effect of substrate mechanical properties
on fibroblast actin cytoskeleton. a–f: NIH 3T3
fibroblasts expressing EGFP-actin were plated on
polyacrylamide gels with rigidities ranging from
180 Pa (a) to 16,000 Pa (f). Fibroblasts on soft
materials had no stress fibers compared with fibro-
blasts on stiffer materials that do have articulated
stress fibers. Scale bar ⫽ 10 m. g,h: NIH 3T3
fibroblasts on soft gels (180 Pa) are fixed with 4%
paraformaldehyde and their F-actin stained with
rhodamine phalloidin. The isolated fibroblast (g)
appears to have no stress fibers as in a. When the
fibroblasts are able to make cell-cell contact (h),
stress fibers form. Scale bar ⫽ 15 m.
GFP-actin, fibroblasts grown on gels softer than 1,600 Pa
A similar dependence of cell shape on stiffness was
had no detectable stress fibers or other actin bundles
also seen with bovine aorta endothelial cells. These cells,
visible by fluorescence microscopy. At stiffnesses above
shown after 1 day in culture in Figure 3, also ranged from
3,600 Pa, such actin fibers were a nearly universal feature
round to well spread as the stiffness was increased about
of these cells.
a few thousand Pa. As with fibroblasts, the round cells on
The absence of stress fibers on soft gels was not an
soft surfaces were capable of division and were able to
indication of toxicity since cell growth rates were only
form confluent monolayers indistinguishable from those
slightly slower on 180-Pa gels compared to the rates on
formed by cells on stiffer surfaces. Figure 3d–f shows
the stiffest gels (55,000 Pa) and rates on gels between
monolayers developed after 3 days of culture on surfaces
1,600 and 3,600 Pa were equal to or greater than those on
of stiffness varying from 180 to 29,000 Pa. Unlike the
the stiffest gels. The absence of stress fibers was also
obvious difference in shape of single cells, these endo-
only evident on single cells on the soft gels. As the cell
thelial cells lose their morphologic difference once they
density increased over time, cells that made cell-cell
make cell-cell contact. The density of cells in the mono-
contact frequently became elongated and developed
layers also was independent of substrate stiffness, with 927,
stress fibers. Figure 2g,h shows two examples of fibro-
898, and 895 adherent cells counted on equal-size areas
blasts grown 48 h on a 180-Pa gel and stained with
(600 ⫻ 490 m) of gels with elastic moduli of 180, 2,900,
rhodamine phalloidin. The single cell (Fig. 2g) shows
and 28,600 Pa, respectively.
phalloidin staining consistent with the images of GFP-
The dependence of cell shape on stiffness is shown
actin under these conditions showing amorphous F-actin
quantitatively in Figure 4. Figure 4a shows the average
distribution and no evidence of stress fibers. Comparable
circumference of 3T3 fibroblasts grown for 1 day on gels of
cells (Fig. 2h) on the same gel stiffness that had made
varying stiffness that were laminated with either fibronectin
intercellular contact show a more flattened morphology
(circles) or type 1 collagen (triangles). The circumference of
and abundant stress fibers.
these cells changes by more than a factor of 4 as the
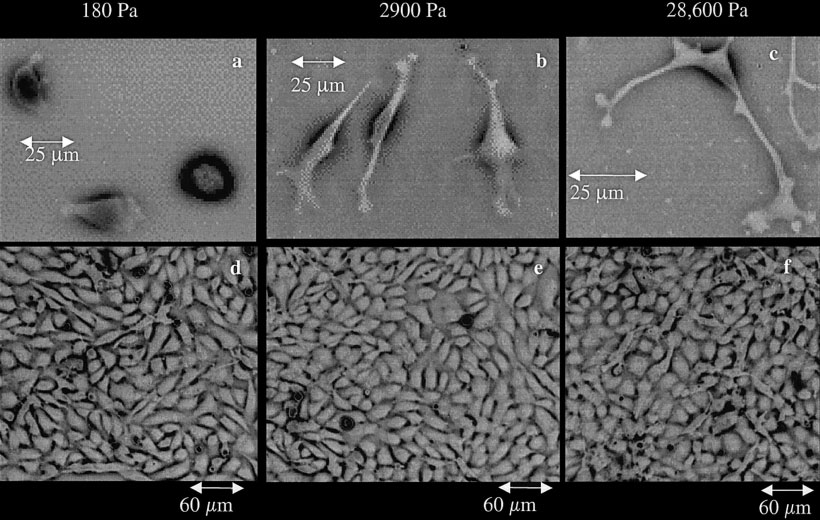
Cell Morphology and Substrate Stiffness
Effect of substrate mechanical properties on endothelial cell morphology. Bovine aortic endo-
thelial cells (BAECs) were plated on polyacrylamide gels. Projected cell area increases with substrate
stiffness (a– c). As cells reach confluence (d–f) a monolayer forms in all groups and the morphologies
become indistinguishable.
substrate stiffness increases and reached a value almost
In contrast to both fibroblasts and endothelial
identical to that on glass for FN-laminated gels with stiff-
cells, human neutrophils exhibit no dependence of cell
ness above 10,000 Pa. On soft gels, 3T3 fibroblasts have
shape on substrate stiffness over the stiffness range
approximately the same size when bound either to FN or
accessible by this system. Figure 4c shows that the
collagen, but on gels with 10,000 Pa, they show more mod-
circumference of neutrophils is constant on gels with
est spreading on collagen than when bound to FN. Similar
stiffness from 2 to 1,000 Pa and equal to that seen on
results were obtained when plotting adherent area instead of
glass. Moreover, the fMLP-stimulated increase in cell
circumference (data not shown). Controls on glass could not
spreading is as large on the softest gel that could be
be done for collagen since in this case FN from the serum-
made (2 Pa) as it is on glass.
containing medium would also bind the glass surface and
The difference in morphology observed after
provide these cells with an additional adhesion protein. The
prolonged incubation on gels with different stiffness
non-monotonic dependence of circumference (or area) with
arises initially from large differences in the rates of
gel stiffness is unexplained but apparently not simply an
spreading after initial adhesion of cells to the surface.
artifact of measuring mean areas of populations with a large
Figure 5 shows time courses of the spreading of two
variance, since in repeated studies using different fibroblast
representative fibroblasts after initial binding to gels
samples and gel preparations, maxima in cell spreading
with stiffness of 180 (Fig. 5a) or 55,000 Pa (Fig. 5b).
were seen at intermediate stiffnesses around 3,000 Pa.
The change in adherent area with time for 5 such cells
Endothelial cells also show a stiffness-dependent
under each condition are shown in Figure 5C and D.
spreading, but the change is not as large as for fibroblasts
There is a rather broad range of spreading rates under
as quantified either by cell circumference (Fig. 4b) or
both conditions, but, on average, cells on soft gels do
area (data not shown). As with fibroblasts, spreading on
not spread fast or very far compared to cells on stiffer
collagen is somewhat less than on FN-coated gels.
gels. On the stiff gels, a few cells fail to spread after
Yeung et al.
Measurement of cell cir-
cumference for different cell types
on substrates of varying rigidities.
NIH 3T3 fibroblasts (a) and BAECs
(b) were plated on flexible substrates
coated with fibronectin (circles) or
type I collagen (triangles) and im-
aged after 24 h in culture. Both fi-
broblasts and BAECs increased in
circumference as substrate stiffness
increased. Cells plated on fibronec-
tin reached a larger apparent circum-
ference than those plated on colla-
gen. A histogram of circumference
measurements (inset in a) shows that
despite a large variation in cell cir-
cumference within a given cell pop-
ulation, the distributions measured at
different stiffnesses overlap very lit-
tle. Human blood neutrophils (c)
were also plated on varying rigidities
and left untreated (open circles) or
stimulated
fMLP. Neutrophil circumference re-mains essentially stable despite gelstiffness. Error bars denote S.E.M.
from measurements of 10 to 200cells for each data point.
initial attachment but most spread at a rate never
(Fig. 6a). The increase in ␣5 integrin expression on
observed on the soft gel. The average rate of spreading
stiff gels may indicate an increase in adhesivity on
as quantified by the initial rate of area versus time
stiffer materials. To determine if ␣5 integrin expres-
(Fig. 5c, inset) is more than 5 times larger on the
sion was sufficient to cause cell spreading, even on
stiffer gel.
soft surfaces, the ␣5 subunit was expressed as an
Coincident with the rather abrupt spreading of
EGFP fusion in NIH 3T3 fibroblasts. The exogenous
fibroblasts on FN-coated surfaces stiffer than 2,000 Pa
expression of EGFP-␣5 had no obvious effect on cell
was a large increase in expression of ␣5 integrin
spreading, as cells on soft and stiff gels followed the
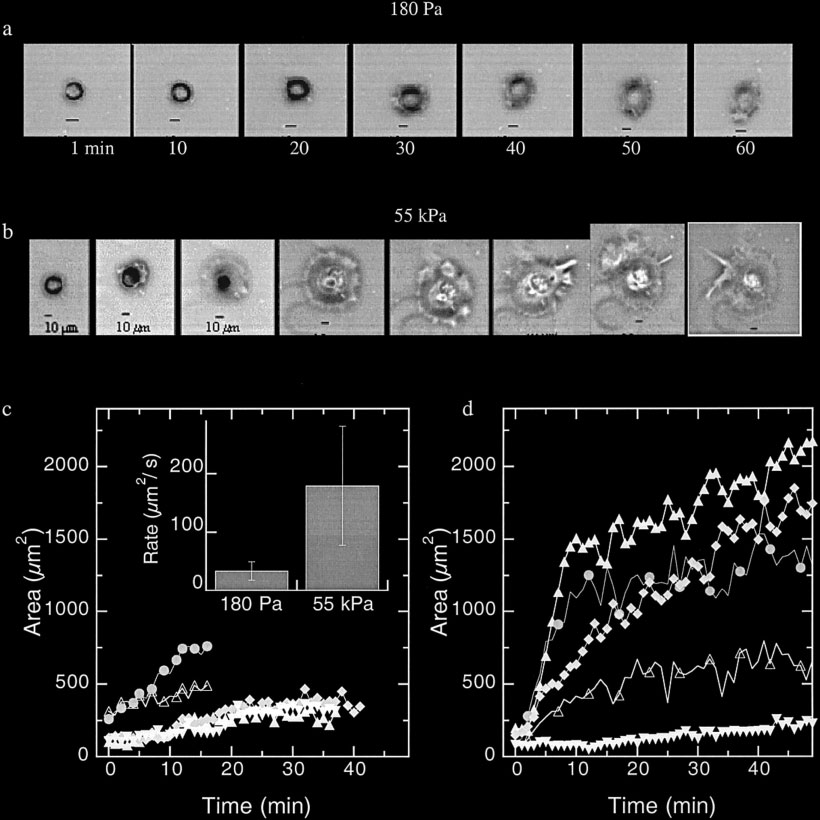
Cell Morphology and Substrate Stiffness
Cell spreading dependence on substrate stiffness. The initial cell spreading as measured by cell
area of NIH3T3 fibroblasts on soft (a,c) and stiff gels (b,d) shortly after plating. a,b: Representative phase
images of fibroblasts spreading on both gels show that cells on soft gels (a) spread very little compared
with the extent of spreading on stiff gels (b). Quantification of adherent cell area shows that cells on soft
gels are smaller and exhibit a slower spreading rate (c) compared to the average of cells on stiff gels (d).
Inset in c: Rate of spreading as quantified by initial rate of area versus time on soft and stiff gels.
same morphological trend as untransfected cells (Fig.
6b,c). On soft gels, ␣5 was evident along the plasmamembrane, but the cell did not spread as it did on stiff
Most cell types in multicellular organisms are at-
gels where the adherent area was larger and the ␣5 had
tached to soft materials, either other cells or extracellular
a punctate distribution.
matrices, but most of what is known about cell structure
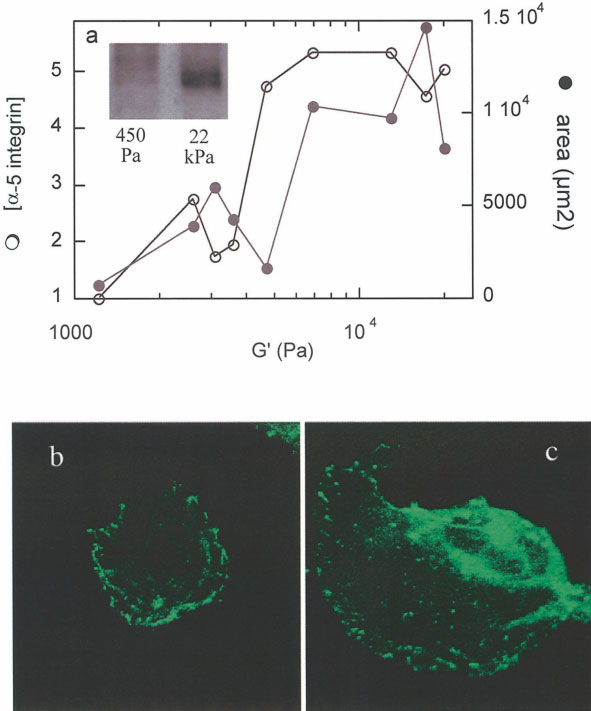
Yeung et al.
Role of ␣5-integrin in fibro-
blasts on flexible substrates. a: Fibro-
blasts plated on varying rigidities were
lysed after 24 h in culture, and ␣5 ex-
pression was determined by Western
blot analysis as shown in the inset. Fi-
broblasts on stiff materials show a 5-fold
increase in protein expression compared
with cells on softer gels. b,c: Exogenous
expression of ␣5 by transfection with
GFP-␣5 had no effect on cell spreading.
Cells on soft gels (b) showed that ␣5
was present and localized to the cell
membrane, but cells remained small and
rounded. In contrast, cells on stiff gels
expressing exogenous ␣5 (c) were well
spread.
and function in vitro derives from studies of cells plated
in different cell types and depends on the nature of the
on rigid substrates such as plastic or glass, laminated
adhesion receptor by which the cell binds its substrate.
with a thin film of protein. As a result, some prominent
There are also important differences between cells grown
aspects of cell structure, such as the fan-shaped morphol-
on two- and three-dimensional adhesive materials, but
ogy of cultured fibroblasts or the prevalence of large
even when confined to adhesion on flat surfaces, the
actin-containing stress fibers that are commonly studied
elastic constant of the surface can determine cell mor-
in vitro, are rarely if ever seen in vivo. One well-recog-
phology and protein expression over a very wide range.
nized and studied reason to account for such differences
Recent studies with aortic smooth muscle cells have
is that many cell types in vivo function within a three-
shown that substrate stiffness is a more important deter-
dimensional matrix, in contrast to the growth of cultured
minant for cell shape than is the density of adhesive
cells at a solid/liquid interface [Bard and Hay, 1975;
ligand to which the cell binds [Engler et al., 2004].
Greenburg and Hay, 1982], but an additional indepen-
In addition to relatively well-recognized mechani-
dent difference relates to differences in elasticity of bio-
cal signals produced at cell-matrix adhesion sites, cells in
logical surfaces [Lo et al., 2000; Pelham and Wang 1997;
mechanically active environments also form extensive,
Wang et al., 2000a]. The studies reported here show that
cadherin-mediated intercellular junctions that are impor-
cellular response to matrix stiffness may be very different
tant in tissue remodeling and differentiation. A relevant
Cell Morphology and Substrate Stiffness
finding in the work reported here is that when endothelial
able to generate large traction forces that it can generate
cells or fibroblasts make cell-cell junctions on soft sub-
during phagocytosis [Evans et al., 1993] and that appear
strates, they convert to the morphology seen on stiffer
to be essential for extension of fibroblast cytoskeletons.
substrates. Specifically, fibroblasts in contact with other
Another example that runs counter to the expectation that
cells spread and develop stress fibers even on surfaces of
cell protrusion requires traction forces exerted on a stiff
100-Pa stiffness, when neighboring single cells maintain
material is observed with neurons derived from embry-
a radically distinct rounded morphology (Fig. 2h). Like-
onic murine spinal cord. These cells extend processes
wise, endothelial cells when confluent have indistin-
that branch most effectively on soft surfaces with maxi-
guishable morphologies on soft and hard substrates while
mal branching on gels with stiffness of 50 Pa [Flanagan
their structures are easily distinguishable when they are
et al., 2002]. The detailed differences in how neutrophils
sparse enough to lack cell-cell junctions.
and other cell types employ actin polymerization and
The simplest explanation for this change is that cells
acto-myosin contractility to move are not known, but
have a binary sensor at their membrane junction sites that
they must be variable enough to allow robust spreading
signals for a relaxed rounded morphology when the surface
and motility of neutrophils and neurons on surfaces that
is softer than the cell's intrinsic elastic modulus, and signals
are far too soft to allow fibroblasts or endothelial cells to
for another phenotype with increased contractility and stress
spread. There are clearly defined differences in the num-
fiber formation when the external material, either another
ber, size, and stability of integrin-based adhesions in
cell or an extracellular matrix, is stiffer than the cell or as
different cell types [Entschladen and Zanker, 2000], and
stiff as the cell itself. An alternate but not exclusive expla-
it seems probable that large focal adhesions characteristic
nation is that the signals for mechanically induced morpho-
of fibroblasts on stiff surfaces do not form nor could they
logic differences come only from extracellular ligand-acti-
function on soft gels [Balaban et al., 2001]. Different cell
vated integrins, and that activated cadherins override these
types may have adapted responses to different ranges of
signals to establish a distinct program. Adherens junctions
stiffness to match their function in vivo.
in connective tissue fibroblasts or other cell types may
The magnitude of stiffness over which fibroblasts
transmit mechanical signals and coordinate multicellular
switch from being round and free of stress fibers to the
adaptations to physical forces [Ko et al., 2001; Nelson and
fan-shaped form with abundant stress fibers seen when
Chen, 2003; Philippova et al., 1998]. The involvement of
grown on rigid surfaces may help differentiate among dif-
cadherin-based adhesions sites in sensing and responding to
ferent possible structures responsible for stiffness sensing.
the mechanical properties of the tissue is strongly suggested
For fibroblasts on fibronectin-coated surfaces, the transition
by the finding that in wound healing, intercellular contacts
occurs around a value for the shear modulus of 1,000 to
involving interactions between cadherins, actin, and myosin
3,000 Pa. This stiffness is similar to the elastic modulus of
are implicated in generating the forces required for wound
the fibroblast itself. Measurements by atomic force micros-
closure [Adams and Nelson, 1998]. Notably, maintenance
copy of Young's modulus (equal to 3 times the shear mod-
of the structure of intercellular contacts critically depends
ulus for simple incompressible solids) of an NIH 3T3 fi-
upon contractile forces generated by the actin cytoskeleton
broblasts on a rigid surface are between 3 and 12 kPa
[Danjo and Gipson 1998] that act on intercellular contacts in
[Rotsch et al., 1999]. The similarity of these elastic con-
adjacent cells [Gloushankova et al., 1998], and the magni-
stants suggests that fibroblasts may undergo spreading only
tude and spatial distribution of these forces depend on the
when internally generated forces, which are reported to be
viscoelastic properties of the cells as well as the underlying
independent of collagen matrix stiffness [Freyman et al.,
2002], are exerted on a surface that is stiff enough so that
One clear outcome of these studies is that not all
the resulting deformation is partly within the cytoskeleton
cells appear to use stiffness as a cue for morphology or
and not only within the external matrix.
motility, and the well-documented increase in spread
The measured shear moduli of the polyacrylamide-
area in fibroblasts [Lo et al., 2000] as matrix stiffness or
based gels used in these studies is somewhat smaller than
density of adhesions sites increases is not universally
the elastic moduli reported from measurements of uniaxial
observed. Figure 4, for example, shows that, unlike fi-
extension or indentation reported in other studies. The
broblasts or endothelial cells, neutrophils appear to be
forces that cells apply to a flat surface are shear forces, and
insensitive to matrix stiffness. In the inactive state, they
therefore the shear modulus measured here is the relevant
are relatively round on all surfaces tested and can spread
parameter quantifying resistance of the material to this
and protrude after stimulation by a chemotactic peptide
geometry of force application. The Young's modulus, as
equally well on a gel with elastic modulus 2 Pa as they do
measured by extensional or indentation methods, is related
on glass. The ability of neutrophils to spread on such a
to the shear modulus by a function that requires knowledge
soft matrix is remarkable because it means that the cell
of the Poisson's ratio of the material. The somewhat smaller
extends its cortical actin-rich periphery without being
values of shear modulus measured directly may help resolve
Yeung et al.
a question related to the mechanism by which cells produce
Engler A, Bacakova L, Newman C, Hategan A, Griffin M, Discher D.
the internal stress that allows them to probe matrix mechan-
2004. Substrate compliance versus ligand density in cell on gel
ical properties. Magnitudes of this stress, reported to be as
responses. Biophys J 86:617– 628.
Entschladen F, Zanker KS. 2000. Locomotion of tumor cells: a mo-
large as 30 kPa in some studies, are difficult to account for
lecular comparison to migrating pre- and postmitotic leuko-
by a purely acto-myosin dependent process because the
cytes. J Cancer Res Clin Oncol 126:671– 681.
concentration of cellular myosin and the known range of
Evans E, Leung A, Zhelev D. 1993. Synchrony of cell spreading and
forces each motor can generate are not sufficient to produce
contraction force as phagocytes engulf large pathogens. J Cell
this level of force per surface area, suggesting that other
Flanagan LA, Ju YE, Marg B, Osterfield M, Janmey PA. 2002. Neurite
force-generating mechanisms such as osmotic stress may be
branching on deformable substrates. Neuroreport 13:2411–2415.
involved. However, the relevant elastic constants may be
Flory P. 1953. Principles of polymer chemistry. Ithaca: Cornell Uni-
smaller than those used to calculate internal stress, and the
versity Press. 672 p.
transition from round to spread morphology of fibroblasts
Freyman TM, Yannas IV, Yokoo R, Gibson LJ. 2002. Fibroblast
shown in Figure 4 suggests that internal stresses of 3,000 Pa
contractile force is independent of the stiffness which resists thecontraction. Exp Cell Res 272:153–162.
may be sufficient for mechanosensing.
Gloushankova NA, Krendel MF, Alieva NO, Bonder EM, Feder HH,
Vasiliev JM, Gelfand IM. 1998. Dynamics of contacts betweenlamellae of fibroblasts: essential role of the actin cytoskeleton.
Proc Natl Acad Sci USA 95:4362– 4367.
The stiffness of the surface to which cells adhere
Greenburg G, Hay ED. 1982. Epithelia suspended in collagen gels can
lose polarity and express characteristics of migrating mesen-
can have a profound effect on cell structure and protein
chymal cells. J Cell Biol 95:333–339.
expression, but these mechanical effects vary with dif-
Ko KS, Arora PD, McCulloch CA. 2001. Cadherins mediate intercel-
ferent cell types, and depend on the nature of the adhe-
lular mechanical signaling in fibroblasts by activation of
sion receptors by which the cells bind their substrate.
stretch-sensitive calcium-permeable channels. J Biol Chem
Fibroblasts and endothelial cells develop a spread mor-
phology and actin stress fibers only when grown on
Laukaitis CM, Webb DJ, Donais K, Horwitz AF. 2001. Differential
dynamics of alpha 5 integrin, paxillin, and alpha-actinin during
surfaces with an elastic modulus greater than 2,000 Pa,
formation and disassembly of adhesions in migrating cells.
with a greater effect seen when bound to fibronectin
J Cell Biol 153:1427–1440.
compared to collagen. In contrast, neutrophils appear to
Lo CM, Wang HB, Dembo M, Wang YL. 2000. Cell movement is
be insensitive to stiffness changes over a very wide
guided by the rigidity of the substrate. Biophys J 79:144 –152.
range. The stiffness-dependence of fibroblasts and endo-
Nelson CM, Chen CS. 2003. VE-cadherin simultaneously stimulates
and inhibits cell proliferation by altering cytoskeletal structure
thelial cells is no longer evident when cells become
and tension. J Cell Sci 116:3571–3581.
confluent, or in the case of fibroblasts even when two
Ory DS, Neugeboren BA, Mulligan RC. 1996. A stable human-derived
cells make contact suggesting either that mechanosens-
packaging cell line for production of high titer retrovirus/
ing uses the cells' internal stiffness as a criterion or else
vesicular stomatitis virus G pseudotypes. Proc Natl Acad Sci
that signaling from cadherins in cell-cell contacts over-
USA 93:11400 –11406.
Pelham RJ, Jr., Wang Y. 1997. Cell locomotion and focal adhesions
rides signals from the cell-matrix adhesion complexes.
are regulated by substrate flexibility. Proc Natl Acad Sci USA94:13661–13665.
Philippova MP, Bochkov VN, Stambolsky DV, Tkachuk VA, Resink
TJ. 1998. T-cadherin and signal-transducing molecules co-
Adams CL, Nelson WJ. 1998. Cytomechanics of cadherin-mediated
localize in caveolin-rich membrane domains of vascular
cell-cell adhesion. Curr Opin Cell Biol 10:572–577.
smooth muscle cells. FEBS Lett 429:207–210.
Balaban NQ, Schwarz US, Riveline D, Goichberg P, Tzur G, Sabanay I,
Rossi FM, Guicherit OM, Spicher A, Kringstein AM, Fatyol K,
Mahalu D, Safran S, Bershadsky A, Addadi L, Geiger B. 2001.
Blakely BT, Blau HM. 1998. Tetracycline-regulatable factors
Force and focal adhesion assembly: a close relationship studied
with distinct dimerization domains allow reversible growth
using elastic micropatterned substrates. Nat Cell Biol 3:466 – 472.
inhibition by p16. Nat Genet 20:389 –393.
Bao G, Suresh S. 2003. Cell and molecular mechanics of biological
Rotsch C, Jacobson K, Radmacher M. 1999. Dimensional and me-
materials. Nat Mater 2:715–725.
chanical dynamics of active and stable edges in motile fibro-
Bard JB, Hay ED. 1975. The behavior of fibroblasts from the devel-
blasts investigated by using atomic force microscopy. Proc Natl
oping avian cornea. Morphology and movement in situ and in
Acad Sci USA 96:921–926.
vitro. J Cell Biol 67:400 – 418.
Wakatsuki T, Kolodney MS, Zahalak GI, Elson EL. 2000. Cell mechanics
Cunningham CC, Vegners R, Bucki R, Funaki M, Korde N, Hartwig
studied by a reconstituted model tissue. Biophys J 79:2353–2368.
JH, Stossel TP, Janmey PA. 2001. Cell permeant polyphosphoi-
Wang HB, Dembo M, Wang YL. 2000a. Substrate flexibility regulates
nositide-binding peptides that block cell motility and actin
growth and apoptosis of normal but not transformed cells. Am J
assembly. J Biol Chem 276:43390 – 43399.
Physiol Cell Physiol 279:C1345–C1350.
Danjo Y, Gipson IK. 1998. Actin "purse string" filaments are anchored
Wang LZ, Gorlin J, Michaud SE, Janmey PA, Goddeau RP, Kuuse R,
by E-cadherin-mediated adherens junctions at the leading edge
Uibo R, Adams D, Sawyer ES. 2000b. Purification of salmon
of the epithelial wound, providing coordinated cell movement.
clotting factors and their use as tissue sealants. Thromb Res
J Cell Sci 111:3323–3332.
Source: http://cytothesis.us/3.0/Oil_Cell-Morphology.pdf
Part 1. Systemic psoriatic process Edition e4.0 Mikhail Peslyak Moscow, 2012 UDC 616.5:616-092 Mikhail Yuryevich Peslyak Model of pathogenesis of psoriasis. Part 1. Systemic psoriatic process. Edition e4.0 (revised and updated), Russia, Moscow, MYPE, 2012.– 84 p. ISBN 978-5-905504-02-0
ASSISTED REPRODUCTIVE TECHNOLOGY PROGRAM PATIENT MANUAL WILLIAM P. HUMMEL, M.D. L. MICHAEL KETTEL, M.D. SAN DIEGO FERTILITY CENTER Making Dreams Come True … IVF PROGRAM Patient Manual WILLIAM P. HUMMEL, M.D. L. MICHAEL KETTEL, M.D.