Human vestibular cortex as identified with caloric stimulation in functional magnetic resonance imaging
NeuroImage
17, 1384 –1393 (2002)
doi:10.1006/nimg.2002.1241
Human Vestibular Cortex as Identified with Caloric Stimulation
in Functional Magnetic Resonance Imaging
Oliver Fasold, Michael von Brevern, Marc Kuhberg, Christoph J. Ploner, Arno Villringer,
Thomas Lempert, and Ru
Department of Neurology, Charite´, Humboldt-University, D-10098 Berlin, Germany
Received March 23, 2002
structures to elucidate vestibular function. Spatial ori-
Anatomic and electrophysiological studies in mon-
entation and perception of movement, however, re-
keys have yielded a detailed map of cortex areas re-
quire processing of vestibular information at the corti-
ceiving vestibular afferents. In contrast, compara-
cal level, but comparatively little is known about the
tively little is known about the cortical representation
cortical representation of the vestibular system. Ani-
of the human vestibular system. In this study we ap-
mal experiments have shown that there is no primary
plied caloric stimulation and fMRI to further charac-
vestibular cortex that obtains projections exclusively
terize human cortical vestibular areas and to test for
from vestibular afferents (Guldin and Gru
¨ sser, 1998).
hemispheric dominance of vestibular information pro-
Instead, several multimodal sensory areas have been
cessing. For caloric vestibular stimulation we used
identified, which integrate vestibular, visual, and so-
cold nitrogen to avoid susceptibility artifacts induced
by water calorics. Right and left side vestibular stim-
matosensory signals (Faugier-Grimaud and Ventre,
ulation was repetitively performed inducing a nystag-
1989; Fredrickson
et al., 1966; Guldin and Gru
mus for at least 90 s after the end of the stimulation in
1998; Odkvist
et al., 1974). In humans, these areas
all subjects. Only the first 60 s of this nystagmus period
have been partly confirmed by intraoperative cortical
was included for statistical analysis and compared
stimulation (Foerster, 1936; Penfield, 1957), by clinical
with the baseline condition. Activation maps revealed
studies in patients with acute cortical lesions (Brandt
a cortical network with right hemispheric dominance,
et al., 1994), and by functional imaging using positron
which in all subjects comprised the temporoparietal
emission tomography (PET) (Bottini
et al., 1994). Pre-
junction extending into the posterior insula and, fur-
vious imaging studies in humans provided evidence
thermore, the anterior insula, pre- and postcentral
that vestibular afferents project predominantly to the
gyrus, areas in the parietal lobe, the ventrolateral por-
right hemisphere but separate stimulation of both ves-
tion of the occipital lobe, and the inferior frontal gyrus
tibular organs has not been performed so far to test for
extending into the inferior part of the precentral sul-
this hypothesis.
cus. In conclusion, caloric stimulation in fMRI reveals
Imaging of the cortical vestibular system with func-
a widespread cortical network involved in vestibular
tional magnetic resonance imaging (fMRI), the most
signal processing corresponding to the findings from
useful tool in functional imaging today, has been diffi-
animal experiments and previous functional imaging
cult to accomplish. Since caloric irrigation of the ears
studies in humans. Furthermore, this study demon-
with hot and cold water is burdened with artifacts due
strates a strong right hemispheric dominance of
vestibular cortex areas regardless of the stimulated
to the paramagnetic properties of water, some authors
side, consistent with the current view of a rightward
have used galvanic (electrical) stimulation of the mas-
asymmetrical cortical network for spatial orientation.
toids (Bucher
et al., 1998; Lobel
et al., 1998, Bense
etal., 2001). Galvanic vestibular stimulation, however,
2002 Elsevier Science (USA)
differs from caloric stimulation in several aspects:
1. In previous fMRI studies, galvanic stimulation has
been applied to both right and left vestibular nervessimultaneously, so that the cortical response to unilat-
For a long time, vestibular research has concen-
eral vestibular activation could not be studied.
trated on labyrinthine, brainstem, and cerebellar
2. Galvanic stimulation evokes primarily ocular tor-
sion, wheras the induced torsional and horizontal nys-
tagmus is rather weak (Zink
et al., 1998) compared
To whom correspondence should be addressed. Fax: ⫹ 49 (89)
2443-26014. E-mail:
[email protected].
with the usual horizontal nystagmus velocity of 10 to
1053-8119/02 $35.00 2002 Elsevier Science (USA)All rights reserved.
HUMAN VESTIBULAR CORTEX
20°/s resulting from caloric stimulation; this corre-
data complemented each experiment. In the first ses-
sponds to a subjective illusion of tilt around the naso-
sion, high-resolution 3D-Flash datasets (TR ⫽ 20 ms,
occipital axis (Bense
et al., 2001) during galvanic stim-
TE ⫽ 5 ms, flip angle ⫽ 30°, field of view ⫽ 256 ⫻ 256
ulation, while caloric stimulation induces a constant
mm, voxel size 1 ⫻ 1 ⫻ 1 mm, 190 slices) were acquired
sensation of rotation.
instead of the MP RAGE to generate an individually
3. With galvanic stimulation probably all branches of
reconstructed and inflated cortical surface as a detailed
the vestibular nerve are activated, originating from
each of the five suborgans of the laryrinth, but it iscontroversial whether galvanic stimulation affects pre-
dominantly otolith afferents (Watson
et al., 1998) or
Caloric stimulation was applied with cold nitrogen
fibers from the semicircular canals (Schneider
et al.,
through a plastic tube into the external ear canal. The
2000). In contrast, caloric stimulation affects primarily
resulting gas temperature was 5–7°C at the end of the
the horizontal semicircular canal.
tube. The flow of nitrogen was adjusted to the individ-
For this study, we implemented caloric vestibular
ual threshold for vestibular sensations, which was
activation in fMRI with gas instead of water to stimu-
identified outside the scanner. Caloric nystagmus was
late each vestibular organ separately. Thereby, we in-
recorded once for each participant outside the scanner
tended to further characterize cortical vestibular areas
with horizontal electro-oculography (EOG) in complete
and to test for hemispheric dominance of vestibular
darkness. Nystagmus slow-phase velocities were mea-
sured by hand from the graph paper by averaging 10representative slow phases during peak intensity of
caloric nystagmus for each direction. Since formerstudies showed considerable interindividual variabil-
ity of cortical activation (Bucher
et al., 1998; Lobel
etal., 1998), we performed an individual analysis of the
Five individuals (four men, one woman, age range
data instead of a group analysis. Each subject under-
24 –36 years) participated in the study; none had a
went 6 to 10 runs comprising two caloric irrigation
history of vestibular or neurological dysfunction. All
cycles of 60 s each for each stimulation side. Functional
subjects were right-handed according to a modified
scans were acquired in complete darkness and partic-
version of the Edinburgh Inventory for Handedness.
ipants had their eyes closed to prevent even minimal
The study was approved by the local ethics committee.
changes of visual input during nystagmus. After the
Informed consent was obtained from each participant
scans, the participants were asked about their percep-
prior to investigation.
tion of motion.
Data Acquisition
Data Analysis
Imaging was performed on a 1.5-T Siemens Vision
Imaging data were analyzed using the Brainvoyager
echo-planar system with a standard head coil. The
3.9 software package (R. Goebel, Max Planck Society,
head was immobilized by vacuum pads to minimize
Germany). The first 4 volumes of each functional run
movement artifacts. Functional scans were acquired in
were discarded to allow for signal equilibration, result-
16 slices covering the supratentorial parts of the brain
ing in a total of 300 volumes for statistical analysis.
except for the orbitofrontal cortex in an oblique orien-
The two-dimensional slice time courses were converted
tation in runs of 304 images per slice using a T2*-
into three-dimensional volume time courses by coreg-
weighted FID echo-planar-imaging sequence (TR ⫽ 2 s,
istration with the three-dimensional anatomical data
TE ⫽ 60 ms, flip angle ⫽ 90°). Slice thickness was set
sets from the same session and interpolation to the
at 5 mm (skip 0.5 mm between slices) with 4 ⫻ 4-mm
resolution of 1 mm3. Coregistration was based on the
in-plane resolution (field of view ⫽ 256 ⫻ 256 mm,
scanner slice position parameters for the functional
imaging matrix ⫽ 64 ⫻ 64). With every functional
and anatomical measurements and was visually con-
session we collected a high-resolution T1-weighted
trolled using the T1 scans, which were taken in the
3D-MP RAGE dataset (TR ⫽ 9.7 ms, TE ⫽ 4 ms, flip
same orientation as the EPI datasets. After 3D-Motion
angle ⫽ 90°, field of view ⫽ 256 ⫻ 256 mm, voxel size
correction, spatially smoothing with a Gaussian kernel
1 ⫻ 1 ⫻ 1 mm, 190 slices) to transform the EPI data
(FWHM ⫽ 2 mm) and linear drift removal, the statis-
into three-dimensional space and to perform statistical
tical maps for the individual subject were computed
analysis from scans that were taken on different days
with the general linear model (GLM). This required a
Z
(four to six sessions per subject). For visual control of
transformation of the time courses prior to multiple
the spatial transformation process a 2D T1-weighted
regression analysis. Since motion perception can in-
scan (TR ⫽ 900 ms, TE ⫽ 14 ms, voxel size 2 ⫻ 2 ⫻ 5
duce compensatory head movements only functional
mm, 16 slices) in the same orientation as the functional
runs that showed a displacement of less than 2 mm in
FASOLD ET AL.
Cortical Areas of the Right Hemisphere Activated in Response to Unilateral Caloric Stimulation
A. Parieto-insular
B. Anterior insula
C. Post. sup. temporal sulcus
D. Post. sup. temporal gyrus
E. Dorsolateral parietal area
F. Inferior temporal sulcus
G. Occipital lateral gyrus
H. Central sulcus
I. Frontoparietal operculum
J. Precentral sulcus
K. Dorsolateral prefrontal
L. Posterior cingular sulcus
M. Anterior cingular gyrus
a Stimulated side.
b (⫹) refers to a BOLD signal increase in fewer than 176 neighboring interpolated voxels at our significance level of
P ⬍ 10⫺9.
either direction before motion correction were in-
No subject reported pain, nausea, sweating, or emo-
cluded. The statistical maps were thresholded at
P ⬍
tional discomfort. Corresponding to the reported self
motion perception EOG recordings revealed a buildup
Three dimensional cortex reconstruction was per-
of nystagmus usually during the last third of the stim-
formed by segmenting and tessellating the gray–white
ulation period. Nystagmus lasted about 90 s with a
matter boundary and by inflating (Carman
et al., 1995;
mean peak slow-phase velocity of 19.8°/s (SD ⫽ 5.0°/s).
Van Essen
et al., 1998) the resulting surface mesh onthe basis of the Flash data. The EPI volume time
BOLD Signal Increases
courses of later sessions were realigned by an auto-matic intensity-based process between the 3D-MP
Regardless of the stimulated side there was a strong
RAGE and the 3D-Flash scan. The statistical maps for
right hemispheric dominance in all subjects with re-
the individual subject of all functional runs could then
spect to the number of areas as well as their cluster
be calculated and overlaid on the individually recon-
size at the chosen statistical threshold. An exception
structed surface.
was the medial surface of each hemisphere. In the
To produce activation maps, baseline measurements
following, clusters activated in at least one hemisphere
were compared with the 60-s period after termination
in at least three subjects are described. The capital
of stimulus application, when the caloric nystagmus
letters in parentheses in this section refer to Tables 1
peaked as confirmed by the EOG measurements out-
and 2 and Figs. 1 and 2.
side the scanner. By excluding the stimulation period
We found a large area of signal increase of
from statistical analysis nonvestibular influences such
the junction of parietal and insular cortex extending
as somatosensory and auditory stimuli were mini-
from the lateral sulcus into the posterior part of the
mized. Between the functional runs we paused for 4
insula (A). A second significant spot of activation was
min to allow the vestibular excitation to subside.
usually situated on the short gyri of the anterior insula(B). These areas could be identified in all participants
(Fig. 2; Tables 1, 2).
Four main foci were ob-
Motion Perception and Nystagmus
served. One was found in the posterior part of the
All subjects reported moderate to strong vestibular
superior temporal sulcus extending into medial tempo-
sensations, which they usually described as being
ral gyrus in some subjects (C). Four participants had a
tilted toward the nonstimulated side. Self-motion per-
significant signal increase on the posterior bank of the
ception started by the end of the caloric stimulation
superior temporal gyrus (D), whereas the dorsolateral
and in all subjects caloric stimulation led to motion
parietal cortex (E) at the junction of the postcentral
perception for about 90 s after the end of the stimulus.
and intraparietal sulci was activated in only three par-
HUMAN VESTIBULAR CORTEX
Cortical Areas of the Left Hemisphere Activated in Response to Unilateral Caloric Stimulation
A. Parieto-insular
B. Anterior insula
C. Post. sup. temporal sulcus
D. Post. sup. temporal gyrus
E. Dorsolateral parietal area
F. Inferior temporal sulcus
G. Occipital lateral gyrus
H. Central sulcus
I. Frontoparietal operculum
J. Precentral sulcus
K. Dorsolateral prefrontal
L. Posterior cingular sulcus
M. Anterior cingular gyrus
a Stimulated side.
b (⫹) refers to a BOLD signal increase in fewer than 176 neighboring interpolated voxels at our significance level of
P ⬍ 10⫺9.
ticipants. Furthermore, four participants showed an
activation focus in the inferior temporal sulcus (F).
Occipital lobe.
Four participants had a significant
Using caloric vestibular stimulation and susceptibil-
spot of activation in the occipital cortex covering the
ity-sensitive fMRI we found activation of a large-scale
anterior portion of the occipital lateral gyrus (G). In the
cortical network with dominance of the right hemi-
fifth participant we also observed activation in this
sphere, irrespective of the stimulated side. We ob-served distinct spots of activation in the parieto-insu-
area, but it failed to reach the cluster size criterion of
lar cortex, around the central sulcus, and in parietal,
temporal, occipital, and frontal areas. In the following
Central region.
Cortical activations were observed
we discuss how these widespread cortical activations
on the pre- and postcentral gyri around the central
can be interpreted with respect to vestibular cortical
maps derived from animal work and previous studies
Frontal lobe.
In the frontal lobe we found three
main foci of BOLD signal increase. One was situated inthe frontoparietal operculum of the inferior frontal gy-
Temporoparietal Junction and Insula
rus extending into the anterior insula in some subjects(I). Additional significant areas could be observed, in
In all subjects activation could be identified at the
the precentral sulcus (J) and in the dorsolateral pre-
temporoparietal junction, which in some subjects ex-
frontal cortex (K).
tended into the posterior insular cortex (for details onvariability of individual activations see Fig. 2). This
Medial surface of the brain.
Activation on the me-
area corresponds closely to the parieto-insular vestib-
dial surface of the brain was found in both hemispheres
ular cortex (PIVC), which has been described in several
in the posterior part of the cingular sulcus (L) and in
monkey species (Gru
¨ sser
et al., 1990; Guldin and
the middle part of cingular gyrus (M). In contrast to the
¨ sser, 1998). PIVC has been postulated to be the
previously described foci a consistent hemispheric
core region within the vestibular cortical system with
dominance could not be observed.
respect to its strong interconnections with other ves-
Variability between the subjects.
Individual recon-
tibular cortex areas including Brodman areas 3aV, 2v,
struction of the cortical surfaces revealed interindi-
and 6, as well as with the vestibular nuclei in the brain
vidual differences in the sulcal and gyral configuration.
stem (Guldin and Gru
¨ sser, 1998). More than 50% of
Variations were also found with respect to the cluster
PIVC neurons receive vestibular input (Guldin and
size at the chosen statistical threshold. For illustra-
¨ sser, 1998). Other neurons of this area respond to
tion, the unfolded right insular cortex is displayed sep-
optokinetic or somatosensory stimuli characterizing
arately for each participant in Fig. 2.
PIVC as a multimodal sensory area (Gru
¨ sser
et al.,
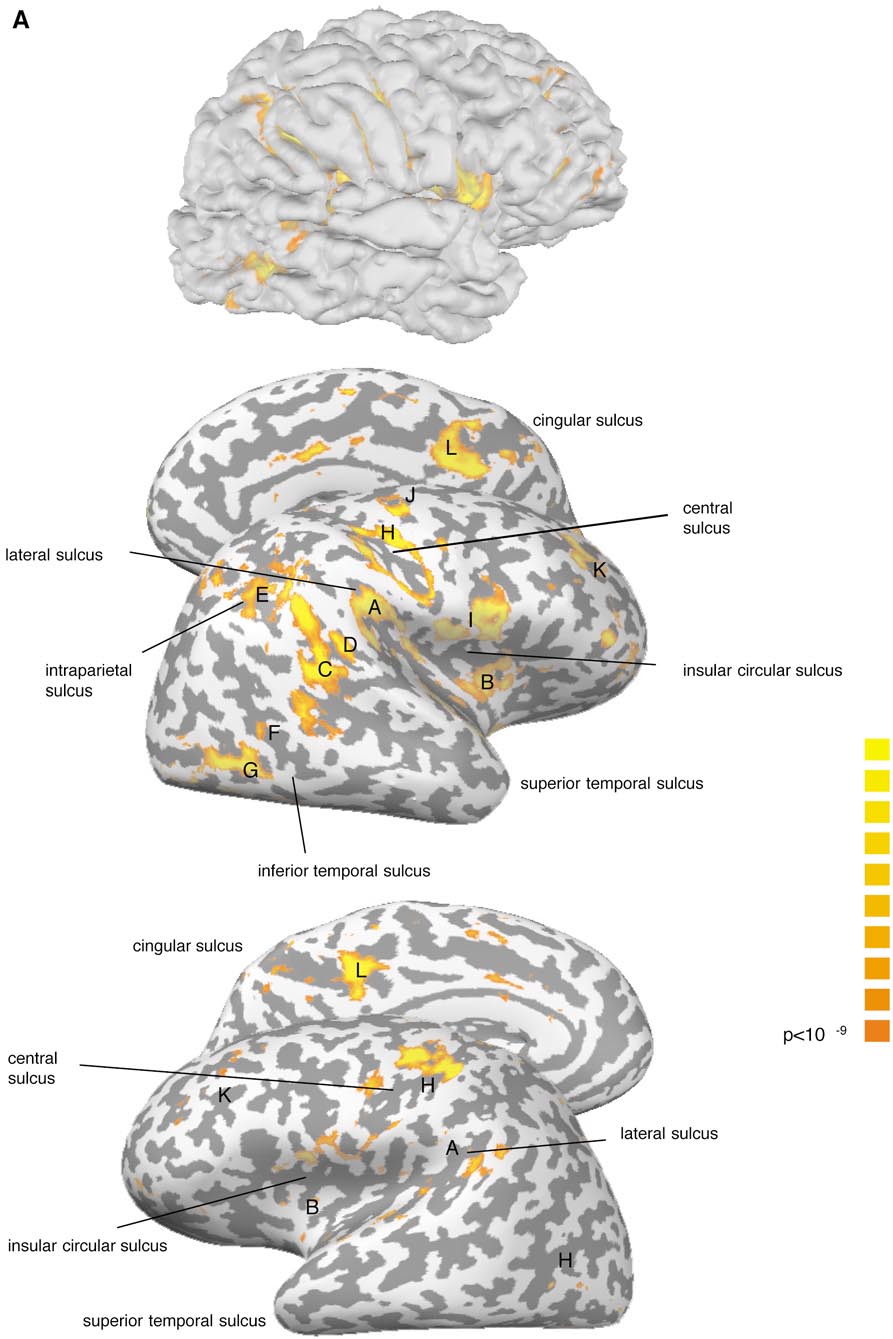
Data of participant a are shown at a threshold of P ⬍ 10⫺9. The light and dark gray stripes symbolize the sulcal and gyral patterns
of the individual unfolded hemisphere, which is shown from lateral and medial perspectives. (A) Vestibular caloric stimulation, left ear; (B)vestibular caloric stimulation, right ear. Upper row: right hemisphere; lower row: left hemisphere.
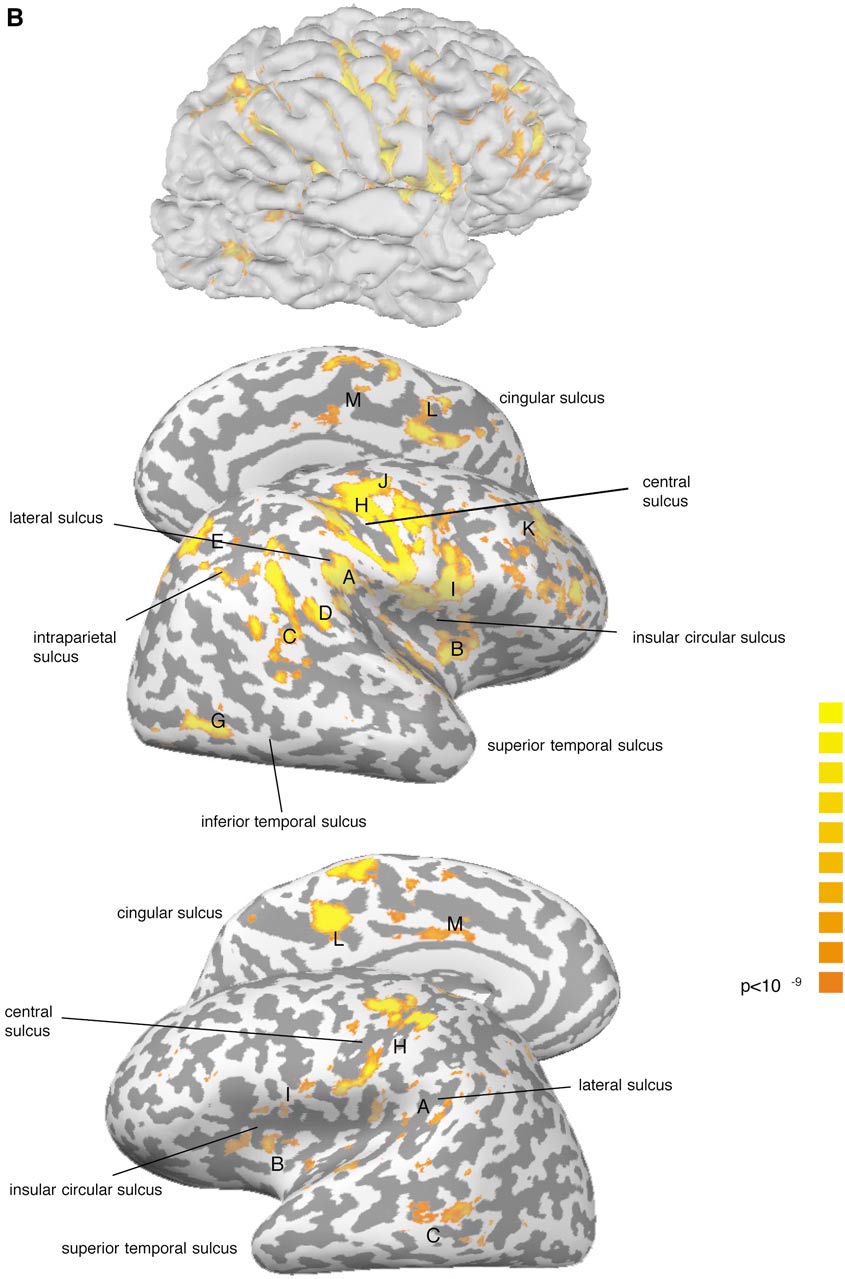
HUMAN VESTIBULAR CORTEX
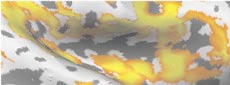
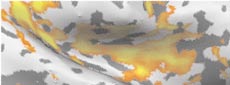
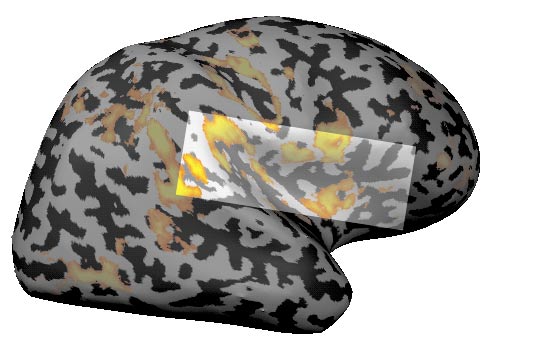
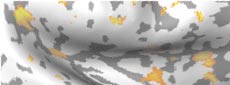
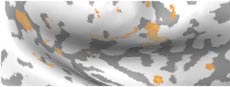
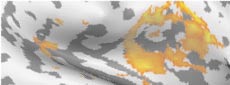
FASOLD ET AL.
Spatial variation of activation in insula region. Data of the right hemisphere are shown for vestibular caloric stimulation of the
left (vcl) and right (vcr) ears thresholded at P ⬍ 10⫺9. For anatomical orientation the insular sulcal pattern is stressed with a dotted line.
Anatomical landmarks: ls, lateral sulcus; stg, superior temporal gyrus; ics, insular circular sulcus; csi, central sulcus of insula.
HUMAN VESTIBULAR CORTEX
1990). Optokinetic activation of PIVC has also been
subjects, we hesitate to do so with respect to the vari-
shown in humans using fMRI (Dieterich et al., 1998).
ance of cortical anatomy between different species. We
Brandt and colleagues (1994) demonstrated that acute
think that further studies are required involving stim-
lesions centered on the posterior insula cause vestibu-
uli from vestibular and other sensory modalities to
lar dysfunction, namely, contraversive tilting of the
clarify the human functional anatomy of these presum-
subjective visual vertical. Our result of PIVC activa-
ably multisensory areas.
tion is further corroborated by previous imaging stud-
Electrical stimulation of the superior temporal gyrus
ies reporting blood flow increases in the temporopari-
in awake humans causes sensations of rotation (Pen-
etal junction and posterior insula as measured by PET
field, 1957). Similarly, focal epileptic seizures arising
during caloric stimulation (Bottini et al., 1994) and
from the posterior temporal lobe may manifest with
during galvanic stimulation (Bucher et al., 1998; Lobel
rotational vertigo and nystagmus (Furman et al.,
et al., 1998, Bense et al., 2001). The interindividual
1990). An early human functional study revealed a
anatomic variation of PIVC (Fig. 2) may account for the
consistent focal activation in the superior temporal
failure to demonstrate PIVC activation in the group
region during vestibular activation (Friberg et al.,
analysis in some galvanic vestibular studies (Dieterich
1985). A recent galvanic study demonstrated activa-
and Brandt, 2000).
tions in the superior temporal gyrus and as well in the
We observed activation not only of the posterior in-
middle temporal gyrus (Bense et al., 2001). Therefore,
sula, but also of the anterior insula in our subjects.
we think that the temporal areas activated in our
While animal studies have failed to demonstrate a
study can be attributed to the human vestibular corti-
vestibular input to this area, the anterior insula can be
cal network.
activated by optokinetic stimulation (Dieterich et al.,1998), which interacts closely with the vestibular sys-
Occipital Lobe
tem. Further, a recent study investigating the human
Interestingly, we observed activation ventrolaterally
neural network of spatial attention demonstrated acti-
in the occipital lobe in the lateral occipital gyrus. In
vations bilaterally in the anterior insula (Kim et al.,
this location an area has been identified as the homo-
1999). The anterior insula is also known to correspond
logue of monkey MT/V5 (Tootell et al., 1995; Zeki et al.,
to both pain and painless thermal stimuli (Davis et al.,
1991). This region is now referred to as hMT/V5 ⫹ or
1998). Although our caloric stimulus was not painful
MT/MST complex since it comprises also the adjacent
and we excluded the stimulation period itself from
area MST, which is closely linked to MT. The principal
statistical analysis to avoid interference with the so-
function of the MT/MST complex is visual motion de-
matosensory and auditory stimulation caused by the
tection and encoding of optic flow. Area MST seems to
air flow, an enduring temperature stimulus can be
receive also semicircular canal signals in primates
assumed. Therefore, activation of the anterior insula
(Bremmer et al., 1999; Thier and Erickson, 1992),
cannot be reliably ascribed to vestibular mechanisms.
which may serve to compensate for head movements inthe encoding of optic flow (Andersen et al., 1999). It is
unlikely that changes in retinal input caused by ves-tibular nystagmus are responsible for MT/MST activa-
The visual posterior sylvian area (VPS) is situated
tion in our participants, since they had their eyes
posterior to PIVC in the inferior parietal lobe in the
closed in darkness inside the scanner.
squirrel monkey. About 30% of the VPS neurons werefound to be vestibularly driven, while most of the neu-
Central Region
rons receive optokinetic or visual input (Guldin andGru
¨ sser, 1998). The neighboring area 7 also receives
Activation around the central sulcus may indicate
vestibular afferents (Faugier-Grimaud and Ventre,
the close link of the vestibular system to the somato-
1989), but vestibularly driven units in this area were
sensory system. Vestibular projections to area 3a have
found to be very rare (Guldin and Gru
¨ sser, 1998). Area
been found in primates. Beside vestibular-driven neu-
2v is another parietal region receiving vestibular affer-
rons in the neck area of area 3a (Akbarian et al., 1994;
ents and is located in the rhesus monkey at the ante-
Guldin et al., 1992) vestibular input to the arm area of
rior end of the intraparietal sulcus (Akbarian et al.,
area 3a has been described (Odkvist et al., 1974).
1994; Fredrickson et al., 1966). In humans the region
Around 40% of the units in the 3a neck region are
around the intraparietal sulcus is also referred to as
driven by vestibular stimuli (Guldin and Gru
parietal eye field and activated during eye movements
1998), and cytoarchitectonically this region may ex-
(Muri et al., 1996). There is evidence that this region is
tend into area 4. Compared with the circumscribed
involved in spatial attention beyond the mere control of
areas reported in the animal literature (Akbarian et
eye movements (Kim et al., 1999). Even if it may be
al., 1994; Guldin et al., 1992; Odkvist et al., 1974) the
tempting to extrapolate the results from monkeys to
extension of the activation spots in this region ap-
activation focuses observed in the parietal lobe of our
peared to be relatively large in some of our subjects.
FASOLD ET AL.
Again, an enduring temperature sensation by the ca-
strong right hemispheric dominance of the activated
loric stimulus, which may have contributed to this
vestibular cortical network. This is consistent with the
activation in the central region, cannot be excluded,
current model of a distributed network for the repre-
although the activations seem to be outside the so-
sentation and exploration of space with hemispheric
matosensory representation field of the ear canal.
dominance on the right (Mesulam, 1981, 1999). Re-cently, functional imaging revealed further experimen-
Frontal Lobe Activations
tal evidence for such an asymmetric large-scale neuralnetwork of spatial attention, which in many aspects
Frontal activations were found in the pars opercu-
overlaps with the vestibular network found in this
laris of the inferior frontal gyrus extending to the in-
study (Kim et al., 1999). Interestingly, vestibular stim-
ferior part of the precentral sulcus. This activation
ulation can transiently reverse impairment of spatial
comprises Brodman area 44 and the caudal part of
exploration in unilateral neglect syndrome (Cappa et
Brodman area 6. Lobel et al. (1998) found activation
al., 1987; Rubens, 1985). In addition to vestibular in-
spots in a similar location in their study with galvanic
put such a network has to integrate and process visual
vestibular stimulation. Presumably these activations
and somatosensory information to construct an inter-
are homologous to the premotor area in monkeys,
nal representation of space (Brandt and Dieterich,
which is interconnected with the PIVC and VPS (Ak-
barian et al., 1993, 1994). In humans an acute right-
Our study has shown that caloric stimulation can be
sided lesion of Brodman area 44 is known to cause
successfully employed in the MRI scanner to visualize
visual neglect (Husain and Kennard, 1996). Activation
vestibular functions at the cortical level. This approach
of the dorsolateral prefrontal cortex, which is involved
may be useful for both physiological and clinical re-
in eye movement control and attentional tasks (Fu-
search as it combines the advantages of unilateral ves-
nahashi et al., 1991) as well as in spatial attention
tibular stimulation with a safe imaging procedure that
(Kim et al., 1999), coincided with activation of the
can be repeatedly applied. In addition, caloric stimula-
frontal eye field (FEF), which is located in the vicinity
tion in fMRI seems to be a particularly sensitive
of the precentral sulcus and in the depth of the caudal-
method for labeling of vestibular areas: this study has
most part of the superior frontal sulcus (Paus, 1996).
shown significant activation of human cortex areas
This activation of the FEF may be associated with
that are probably homologous to the major cortical
nystagmus, although a vestibular nystagmus does not
areas that have been found to receive vestibular input
depend on involvement of the FEF. Such activation
in several primate species (Guldin and Gru
¨ sser, 1998).
either may reflect a feedback signal encoding eye posi-
In addition, our approach allowed us to explore system-
tion during vestibular nystagmus or, beyond mere oc-
atically the laterality of the vestibular cortical net-
ulomotor control, may indicate the involvement of the
work, revealing a strong right hemispheric dominance.
network of spatial attention with its cortical epicenterslocated in the posterior parietal cortex, the frontal eyefields, and the cingulate gyrus (Mesulam, 1999).
Cingular Gyrus Activations
Support was provided by the Deutsche Forschungsgemeinschaft
(Klinische Forschergruppe der Neurologischen Klinik der Charite´
Both the anterior and posterior parts of the cingular
and Grant LE 603/4-1). Oliver Fasold was supported by the graduate
gyrus were activated during vestibular-stimulation. In
program of the Deutsche Forschungsgemeinschaft.
the squirrel monkey corticocortical connections of theso-called "inner cortical vestibular circuit" comprising
PIVC, 3aV, and area 2 to the anterior cingulate cortexhave been shown (Guldin et al., 1992). Parts of thecingulate cortex participate in the cortical network
Akbarian, S., Gru
¨ sser, O. J., and Guldin, W. O. 1993. Corticofugal
projections to the vestibular nuclei in squirrel monkeys: further
monitoring head and body movements in space. A sim-
evidence of multiple cortical vestibular fields. J. Comp. Neurol.
ilar role in spatial and particularly visuospatial atten-
332: 89 –104.
tion has been established in humans (Corbetta et al.,
Akbarian, S., Gru
¨ sser, O. J., and Guldin, W. O. 1994. Corticofugal
1993; Kim et al., 1999; Mesulam, 1999).
connections between the cerebral cortex and brainstem vestibular
nuclei in the macaque monkey. J. Comp. Neurol. 339: 421– 437.
Asymmetries of Vestibular Activation
Andersen, R. A., Shenoy, K. V., Snyder, L. H., Bradley, D. C., and
Crowell, J. A. 1999. The contributions of vestibular signals to the
Unlike previous investigators, we applied unilateral
representations of space in the posterior parietal cortex. Ann. NY
Acad. Sci. 871: 282–292.
vestibular stimulation on either side in all partici-
Bense, S., Stephan, T., Yousry, T. A., Brandt, T., and Dieterich, M.
pants, which allowed insight into the hemispheric spe-
2001. Multisensory cortical signal increases and decreases during
cialization of vestibular information processing. Re-
vestibular galvanic stimulation (fMRI). J. Neurophysiol. 85: 886 –
gardless of the stimulated side we found a reproducible
HUMAN VESTIBULAR CORTEX
Bottini, G., Sterzi, R., Paulesu, E., Vallar, G., Cappa, S. F., Erminio,
Guldin, W. O., Akbarian, S., and Gru
¨ sser, O. J. 1992. Cortico-cortical
F., Passingham, R. E., Passingham, R. E., Frith, C. D., and Frack-
connections and cytoarchitectonics of the primate vestibular cor-
owiak, R. S. J. 1994. Identification of the central vestibular pro-
tex: A study in squirrel monkeys (Saimiri sciureus). J. Comp.
jections in man: A positron emission tomography activation study.
Neurol. 326: 375– 401.
Exp. Brain Res. 99: 164 –169.
Husain, M., and Kennard, C. 1996. Visual neglect associated with
Brandt, T., and Dieterich, M. 1999. The vestibular cortex: Its loca-
frontal lobe infarction. J. Neurol. 243: 652– 657.
tions, functions, and disorders. Ann. NY Acad. Sci. 871: 293–312.
Kim, Y. H., Gitelman, D. R., Nobre, A. C., Parrish, T. B., LaBar, K. S.,
Brandt, T., Dieterich, M., and Danek, A. 1994. Vestibular cortex
and Mesulam, M. M. 1999. The large-scale neural network for
lesions affect the perception of verticality. Ann. Neurol. 35: 403–
spatial attention displays multifunctional overlap but differential
asymmetry. NeuroImage 9: 269 –277.
Bremmer, F., Kubischik, M., Pekel, M., Lappe, M., and Hoffmann,
Lobel, E., Kleine, J. F., Bihan, D. L., Leroy-Willig, A., and Berthoz, A.
K. P. 1999. Linear vestibular self-motion signals in monkey medial
1998. Functional MRI of galvanic vestibular stimulation. J. Neu-
superior temporal area. Ann. NY Acad. Sci. 871: 272–281.
rophysiol. 80: 2699 –2709.
Bucher, S. F., Dieterich, M., Wiesmann, M., Weiss, A., Zink, R.,
Mesulam, M. M. 1981. A cortical network for directed attention and
Yousry, T. A., and Brandt, T. 1998. Cerebral functional magnetic
unilateral neglect. Ann. Neurol. 10: 309 –325.
resonance imaging of vestibular, auditory, and nociceptive areas
Mesulam, M. M. 1999. Spatial attention and neglect: Parietal, fron-
during galvanic stimulation. Ann. Neurol. 44: 120 –125.
tal and cingulate contributions to the mental representation and
Cappa, S., Sterzi, R., Vallar, G., and Bisiach, E. 1987. Remission of
attentional targeting of salient extrapersonal events. Philos.
hemineglect and anosognosia during vestibular stimulation. Neu-
Trans. R. Soc. London B. 354: 1325–1346.
ropsychologia 25: 775–782.
Muri, R. M., Iba-Zizen, M. T., Derosier, C., Cabanis, E. A., and
Carman, G. J., Drury, H. A., and Van Essen, D. C. 1995. Computa-
Pierrot-Deseilligny, C. 1996. Location of the human posterior eye
tional methods for reconstructing and unfolding the cerebral cor-
field with functional magnetic resonance imaging. J. Neurol. Neu-
tex. Cereb. Cortex 5: 506 –517.
rosurg. Psychiatry 60: 445– 448.
Corbetta, M., Miezin, F. M., Shulman, G. L., and Petersen, S. E.
Odkvist, L. M., Schwarz, D. W., Fredrickson, J. M., and Hassler, R.
1993. A PET study of visuospatial attention. J. Neurosci 13: 1202–
1974. Projection of the vestibular nerve to the area 3a arm field in
the squirrel monkey (Saimiri sciureus). Exp. Brain Res. 21: 97–
Davis, K. D., Kwan, C. L., Crawley, A. P., and Mikulis, D. J. 1998.
Functional MRI study of thalamic and cortical activations evoked
Paus, T. 1996. Location and function of the human frontal eye-field:
by cutaneous heat, cold, and tactile stimuli. J. Neurophysiol. 80:
A selective review. Neuropsychologia 34: 475– 483.
Penfield, W. 1957. Vestibular sensation and the cerebral cortex. Ann.
Dieterich, M., and Brandt, T. 2000. Brain activation studies on
Otol. Rhinol. Laryngol. 66: 691– 698.
visual-vestibular and ocular motor interaction. Curr. Opin. Neu-
Rubens, A. B. 1985. Caloric stimulation and unilateral visual ne-
rol. 13: 13–18.
glect. Neurology 35: 1019 –1024.
Dieterich, M., Bucher, S. F., Seelos, K. C., and Brandt, T. 1998.
Schneider, E., Glasauer, S., and Dieterich, M. 2000. Central process-
Horizontal or vertical optokinetic stimulation activates visual mo-
ing of human ocular torsion analyzed by galvanic vestibular stim-
tion-sensitive, ocular motor and vestibular cortex areas with right
ulation. NeuroReport 11: 1559 –1563.
hemispheric dominance: An fMRI study. Brain 121: 1479 –1495.
Thier, P., and Erickson, R. G. 1992. Vestibular input to visual-
Faugier-Grimaud, S., and Ventre, U. 1989. Anatomic connections of
tracking neurons in area MST of awake rhesus monkeys. Ann. NY
inferior parietal cortex (area 7) with subcortical structures related
Acad. Sci. 656: 960 –963.
to vestibulo-ocular function in a monkey (Macaca fascicularis).
Tootell, R. B., Reppas, J. B., Kwong, K. K., Malach, R., Born, R. T.,
J. Comp. Neurol. 280: 1–14.
Brady T. J., Rosen, B. R., and Belliveau, J. W. 1995. Functional
Foerster, O. 1936. In Sensible cortikale Felder (O. Bumke and O.
analysis of human MT and related visual cortical areas using
Foerster, Eds.), pp. 358 – 449. Springer-Verlag, Berlin.
magnetic resonance imaging. J. Neurosci. 15: 3215–3230.
Fredrickson, J. M., Scheid, P., Figge, U., and Kornhuber, H. H. 1966.
Van Essen, D. C., Drury, H. A., Joshi, S., and Miller, M. I. 1998.
Vestibular nerve projection to the cerebral cortex of the rhesus
Functional and structural mapping of human cerebral cortex: So-
monkey. Exp. Brain Res. 2: 318 –327.
lutions are in the surfaces. Proc. Natl. Acad. Sci. USA 95: 788 –
Friberg, L., Olsen, T. S., Roland, P. E., Paulson, O. B., and Lassen,
N. A. 1985. Focal increase of blood flow in the cerebral cortex of
Watson, S. R. D., Brizuela, A. E., Curthoys, I. S., Colebatch, J. G.,
man during vestibular stimulation. Brain 108: 609 – 623.
Colebatch, H. G., MacDougall, H. G., and Halmaghyi, G. M. 1998.
Funahashi, S., Bruce, C. J., and Goldman-Rakic, P. S. 1991. Neuro-
Maintained ocular torsion produced by bilateral and unilateral
nal activity related to saccadic eye movements in the monkey's
galvanic (DC) vestibular stimulation in humans. Exp. Brain Res.
dorsolateral prefrontal cortex. J. Neurophysiol. 65: 1464 –1483.
122: 453– 458.
Furman, J. M., Crumrine, P. K., and Reinmuth, O. M. 1990. Epileptic
Zeki, S., Watson, J. D., Lueck, C. J., Friston, K. J., Kennard, C., and
nystagmus. Ann. Neurol. 27: 686 – 688.
Frackowiak, R. S. 1991. A direct demonstration of functional spe-
¨ sser, O. J., Pause, M., and Schreiter, U. 1990. Localization and
cialization in human visual cortex. J. Neurosci. 11: 641– 649.
responses of neurones in the parieto-insular vestibular cortex of
Zink, R., Bucher, S. F., Weiss, A., Brandt, T., and Dieterich, M. 1998.
awake monkeys (Macaca fascicularis). J. Physiol. 430: 537–557.
Effects of galvanic vestibular stimulation on otolithic and semicir-
Guldin, W. O., and Gru
¨ sser, O. J. 1998. Is there a vestibular cortex?
cular canal eye movements and perceived vertical. Electroencepha-
Trends. Neurosci. 21: 254 –259.
logr. Clin. Neurophysiol. 107: 200 –205.
Source: http://hsinnamon.web.wesleyan.edu/wescourses/NSB-Psyc275/NeuroAnatomical%20Stories/Vestibular%20Cortex/Fasold.pdf
Texte rédigé par Caroline Sirois, Texte original soumis Révision: Mirella Faubert, B. Pharm., M.Sc., B. Pharm., M.Sc., Ph.D., professeure, pharmacienne à la pharmacie Guy Desjardins, Département des sciences infirmières, UQAR Marc-André Lemieux et Danielle Pelletier, Lévis. Texte final remis le 25 avril 2014 L'auteure et la réviseure scientifique ne déclarent aucun conflit d'intérêts lié à la rédaction de cet article.
The Use and Misuse ofAntibiotics in UK Agriculture Part 1: Current Usage by John Harvey and Liz Mason with a preface by Peter Luff MP £15.00 (Soil Association members, £10.00) 40-56 Victoria Street T 0117 929 0661 F 0117 925 2504 Published Dec 1998 Preface by Peter Luff MP The Soil Association's recommendations Introduction and analysis by Richard Young, Soil Association Campaigns and Policy Coordinator